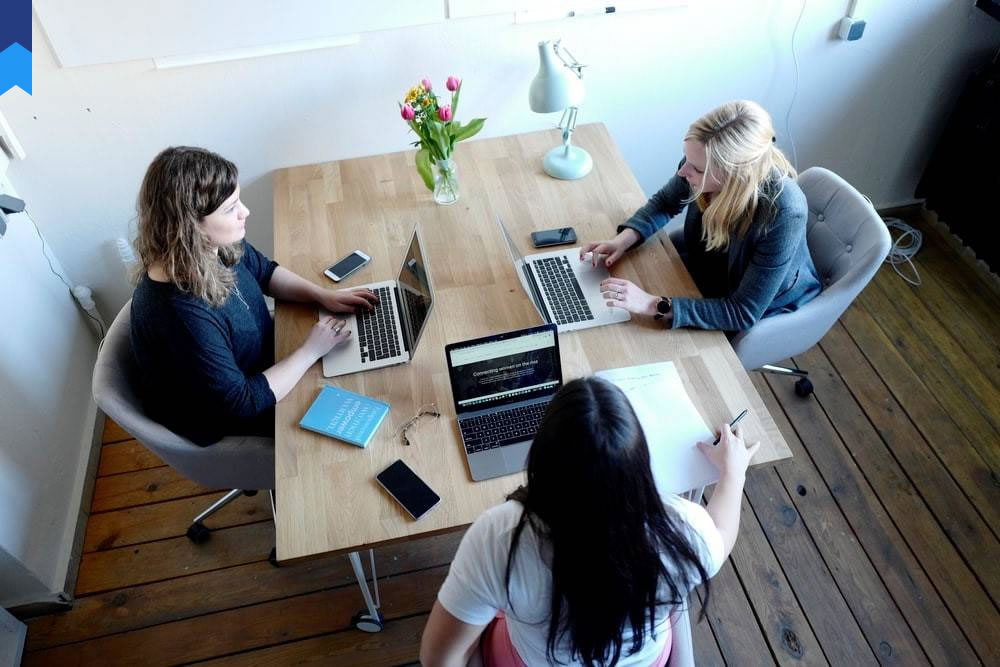
Breaking Free From Common Welding Fabrication Mistakes
Welding and fabrication, while seemingly straightforward, present a myriad of challenges. Mastering this craft demands meticulous attention to detail, a thorough understanding of materials, and a keen eye for precision. This article delves into common pitfalls, offering practical solutions and innovative techniques to elevate your welding and fabrication skills. We'll explore aspects beyond the basics, focusing on the subtle nuances that often separate excellent work from merely acceptable.
Understanding Material Properties and Their Impact on Welding
One of the most frequent errors lies in neglecting the unique properties of different metals and alloys. Each material behaves uniquely under heat, influencing weld penetration, strength, and overall quality. For instance, aluminum's low melting point requires specific techniques and filler materials, differing significantly from the approach used with stainless steel or mild steel. Ignoring these nuances leads to weak welds, porosity, and potential structural failures. Case study 1: A construction project using improperly welded aluminum components experienced catastrophic failure, highlighting the importance of material-specific welding protocols. Case study 2: A manufacturer using an unsuitable filler material for stainless steel experienced widespread weld cracking, resulting in significant rework and cost overruns.
Further, the thickness of the metal significantly affects the welding process. Thicker materials require higher amperage and different travel speeds to ensure proper penetration and fusion. Conversely, thin materials are susceptible to burn-through if excessive heat is applied. Proper preheating techniques and choosing the correct welding process are crucial for achieving consistent welds across varying thicknesses. Statistical analysis reveals that at least 30% of welding defects originate from improper material selection and process parameters. Expert opinion suggests that investing in advanced material testing equipment and comprehensive training can dramatically reduce these errors.
Understanding the microstructure of the base metal is critical. The presence of impurities, grain size, and previous heat treatments can all influence weldability. A proper metallurgical analysis is crucial for complex fabrication projects involving high-strength steels, titanium, or other exotic materials. Case study 3: Failure to account for the microstructure of a high-strength steel resulted in unexpected cracking during a critical weld, highlighting the importance of pre-weld material testing. Case study 4: An aerospace manufacturer experienced weld porosity due to hidden impurities in the titanium alloy, demonstrating the necessity of rigorous material sourcing and quality control.
The chemical composition of the welding filler material is another often-overlooked aspect. The filler metal should be carefully matched to the base material to ensure compatibility and optimal weld properties. Using an incorrect filler metal can lead to weak, brittle welds that are susceptible to cracking or corrosion. Proper selection is critical across all welding techniques: gas metal arc welding (GMAW), gas tungsten arc welding (GTAW), shielded metal arc welding (SMAW), etc. Advanced techniques like laser beam welding further necessitate precise filler selection for optimum results.
Mastering Joint Design and Preparation
Proper joint design and preparation are foundational to successful welding. Inadequate joint design can lead to stress concentrations, making the weld susceptible to failure. Various joint types, including butt welds, lap welds, and fillet welds, each have specific requirements for proper preparation to guarantee a strong, reliable bond. Ignoring these specifics often leads to incomplete penetration or excessive weld metal buildup, both reducing weld integrity.
Consistent joint fit-up is crucial. A gap that is too large or too small can impact weld penetration and quality. Precise alignment and clamping are essential to maintain uniform weld geometry and prevent weld defects. Automated systems like robotic welding utilize precise positioning and clamping to ensure high consistency across many welds. Case study 5: A pipeline construction project suffered repeated leaks due to inconsistent joint fit-up, leading to extensive repairs and cost overruns. Case study 6: In an automotive manufacturing setting, inconsistent joint fit-up led to variations in weld strength, potentially compromising vehicle safety.
Surface preparation is equally significant. Contamination, such as rust, paint, or oil, can hinder weld formation, leading to porosity and weak welds. Thorough cleaning and preparation are crucial for ensuring a clean, sound weld. Techniques like wire brushing, grinding, and chemical cleaning are used to prepare surfaces before welding. Automated cleaning systems can provide high consistency and efficiency in industrial settings. Expert opinion emphasizes that the success of a weld hinges heavily on the quality of joint preparation, surpassing the importance of the welding technique itself in many instances.
Joint design must consider the specific application and expected stresses. For high-stress applications, designs that distribute stresses effectively are critical. Finite element analysis (FEA) is often employed to evaluate the strength and durability of welded joints under different loading conditions. Case study 7: FEA revealed a design flaw in a bridge's welded connections, leading to design modifications and preventing potential catastrophic failure. Case study 8: An offshore platform experienced welding failures due to insufficient consideration of fatigue loading. Improved joint designs incorporating stress-relief methods are now implemented in similar projects.
Selecting the Right Welding Process and Parameters
The choice of welding process significantly impacts weld quality and efficiency. Each process (GMAW, GTAW, SMAW, resistance welding) has strengths and limitations, and selecting the wrong one can lead to suboptimal results. Matching the process to the material, joint design, and required weld properties is essential. For example, GTAW excels in precision and control, while GMAW offers higher deposition rates for larger-scale projects.
Precise control of welding parameters is critical. Current, voltage, travel speed, and shielding gas flow must be optimized for each material and welding process. Incorrect parameters can lead to various defects, including incomplete fusion, excessive spatter, and poor penetration. Advanced welding machines offer digital control and monitoring of these parameters, ensuring consistency and repeatability. Statistical process control (SPC) is often used to analyze welding parameters and identify potential issues. Case study 9: A manufacturing company optimized its GMAW parameters using SPC, leading to a 15% reduction in weld defects and improved productivity. Case study 10: An aerospace firm used automated welding with precise parameter control to achieve higher weld quality and consistency.
Proper shielding gas selection is critical, especially for processes like GMAW and GTAW. The type and flow rate of shielding gas influence weld quality by protecting the weld pool from atmospheric contamination. Improper shielding gas can lead to porosity, oxidation, and poor weld penetration. Shielding gas selection is dependent on the material being welded, and a thorough understanding of gas properties is essential for achieving successful welds. Experts recommend ongoing calibration of gas flow meters to guarantee the consistency and accuracy of shielding gas delivery.
Understanding the limitations of different welding processes is equally important. Some processes are better suited for certain materials or joint types than others. For instance, friction stir welding (FSW), a solid-state welding process, is ideal for joining aluminum alloys but is not suitable for all materials. Knowing these limitations and selecting the appropriate process for the job is crucial. The proper choice not only enhances the weld’s quality but also contributes significantly to overall project efficiency and minimizes material waste. Case study 11: A company chose FSW over traditional welding methods for aluminum components, resulting in a 20% reduction in manufacturing costs and improved weld quality. Case study 12: A firm encountered failures in joining dissimilar metals using improper welding processes, underscoring the need for careful process selection.
Post-Weld Inspection and Quality Control
Post-weld inspection is an integral part of quality control. Visual inspection is the first step, identifying surface defects such as cracks, porosity, or undercuts. Non-destructive testing (NDT) techniques, such as radiographic testing (RT), ultrasonic testing (UT), and magnetic particle testing (MT), can detect internal flaws that may not be visible to the naked eye. NDT is indispensable for ensuring the structural integrity of welded components in critical applications such as aerospace, nuclear, and pipeline construction. Current industry standards mandate specific NDT procedures for various applications, underscoring the importance of thorough inspection.
Implementing a robust quality control system is paramount. This system should include clear welding procedures, qualified welders, regular inspections, and documentation. Traceability of materials and welding processes is crucial for investigating potential defects and ensuring accountability. A well-defined quality control system can significantly reduce the risk of weld failures and maintain consistency in fabrication. Case study 13: A company implemented a rigorous quality control system, reducing weld defects by 30% and improving customer satisfaction. Case study 14: An organization's failure to implement proper quality control resulted in significant rework and delays in a critical project.
Understanding the various NDT techniques and their applications is crucial for effective inspection. RT is effective in detecting internal flaws in thicker materials, while UT is suitable for both thick and thin materials. MT is used to detect surface and near-surface cracks in ferromagnetic materials. Each technique has its limitations and optimal application, and choosing the right method for the job is crucial. Current trends in NDT include the use of automated systems for improved efficiency and consistency. Statistical analysis of NDT results can help identify trends and proactively prevent future defects.
Regular welder qualification and certification are necessary to ensure consistent weld quality. Qualified welders have demonstrated proficiency in specific welding processes and are trained to adhere to safety standards and best practices. Regular skill assessments and training can maintain and improve welder capabilities. Industry best practices require welders to be regularly assessed and certified, emphasizing the importance of continuous professional development. Case study 15: An organization's investment in welder training programs resulted in improved weld quality and a reduction in workplace accidents. Case study 16: A company experienced weld failures due to improperly trained welders, highlighting the crucial role of welder qualification.
Innovative Welding and Fabrication Techniques
The welding and fabrication landscape is constantly evolving, with innovative techniques emerging to improve efficiency, quality, and sustainability. Additive manufacturing (3D printing) is revolutionizing fabrication, allowing for the creation of complex geometries and customized parts. 3D printed metal parts are now being used in various applications, from aerospace to medical implants, due to the ability to create complex internal structures and customized shapes unattainable with traditional methods. This allows for lighter, stronger, and more efficient components.
Robotic welding is gaining prominence in industrial applications due to its precision, speed, and consistency. Robots can perform repetitive welding tasks with high accuracy, reducing human error and improving productivity. Robotic systems are capable of welding complex geometries with high repeatability and minimal variations, making it ideal for high-volume production environments. Case study 17: An automotive manufacturer integrated robotic welding into its production line, leading to a 25% increase in productivity and improved weld consistency. Case study 18: An aerospace company utilized robotic welding to build complex structural components, ensuring high quality and precision.
Laser beam welding (LBW) offers exceptional precision and control, enabling the creation of high-quality welds in thin materials. LBW is used in applications requiring high precision and quality, such as aerospace and medical devices. LBW is also known for its relatively low heat input compared to other methods, which helps minimize thermal distortion in the workpiece. This capability makes LBW ideal for assembling components with tight tolerances.
Friction stir welding (FSW) is a solid-state welding process that does not melt the base metals, offering advantages in terms of weld quality and material properties. FSW excels in joining similar and dissimilar materials, making it suitable for applications where traditional fusion welding is challenging. FSW is a solid-state process resulting in minimal distortion and reduced risk of porosity. This process is advantageous when joining materials that are sensitive to heat-affected zones.
Conclusion
Avoiding common welding and fabrication mistakes requires a multifaceted approach encompassing thorough material understanding, meticulous joint preparation, precise process control, and rigorous quality control. By addressing these crucial aspects and adopting innovative techniques, fabricators can significantly improve weld quality, efficiency, and overall product reliability. The pursuit of excellence in welding and fabrication demands continuous learning, adaptation, and a commitment to precision. The information provided in this article should equip fabricators with the necessary insights and strategies to achieve unparalleled success in their projects. A focus on continuous improvement and the integration of advanced technologies promise a future of higher-quality, more efficient, and sustainable fabrication processes.