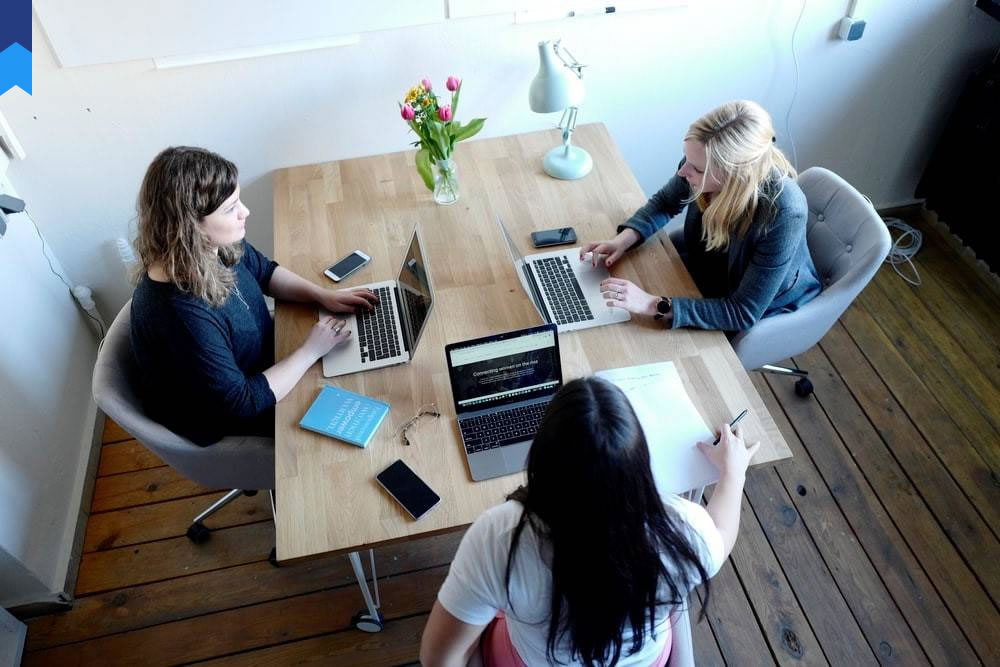
Beyond Traditional Audio: Immersive Soundscapes
Immersive audio is rapidly transforming the way we experience sound, moving beyond traditional stereo and offering new possibilities for entertainment, communication, and even therapy. This exploration delves into the practical applications and innovative advancements of this transformative technology.
Spatial Audio: Redefining Listening Experiences
Spatial audio, a cornerstone of immersive sound, creates a three-dimensional soundscape, enveloping the listener in a realistic auditory environment. Unlike traditional stereo, which presents sound from two channels, spatial audio utilizes multiple channels to precisely position sounds in space. This allows for a much more realistic and engaging listening experience. Consider the impact on gaming, where the ability to pinpoint the location of enemy footsteps or distant gunfire drastically enhances gameplay immersion. Similarly, in virtual reality (VR), spatial audio is crucial for creating believable and interactive environments. Imagine a VR concert experience where the sounds of the instruments emanate from specific locations on a virtual stage, mirroring a live performance. The technology behind spatial audio is constantly evolving, with advancements in head tracking and binaural rendering further enhancing its realism.
One prime example of spatial audio's application is the use of Dolby Atmos in home theater systems. This technology leverages object-based audio, allowing sound engineers to place individual sounds within a three-dimensional space, creating a rich and detailed soundscape. Another example is the implementation of spatial audio in headphones. Many modern headphones now incorporate sophisticated algorithms to simulate a surround sound experience, even in a personal listening setting. These advancements are pushing the boundaries of what’s possible in audio production and consumption, setting new standards for immersive entertainment.
Furthermore, the development of advanced algorithms and processing power continues to refine spatial audio experiences. Real-time rendering and dynamic head tracking are key components that enhance realism. Real-time rendering ensures that sound changes dynamically based on the listener's movement, while head tracking ensures the sound is accurately positioned relative to the listener's orientation. This creates a uniquely personal and immersive listening experience, unlike anything previously possible. Case studies show that users consistently report increased levels of engagement and immersion with spatial audio. For instance, research has demonstrated improved emotional response to music and enhanced focus in gaming environments due to the increased realism provided by spatial audio technologies.
The future of spatial audio is bright, with continued advancements in technology promising even more realistic and immersive listening experiences. The integration of artificial intelligence (AI) could further personalize these experiences by adapting sound profiles to individual listeners. Moreover, advancements in haptic feedback technology could add another dimension of physical sensation to spatial audio, providing a truly multi-sensory listening experience that goes beyond mere sound.
Sonification: Giving Data a Voice
Sonification, the process of transforming data into sound, provides a novel and often more intuitive way to understand complex information. Instead of relying solely on visual representations, sonification uses auditory cues to convey data patterns and relationships. Imagine tracking stock market fluctuations through a rising and falling melody, or visualizing astronomical data as a celestial soundscape. Sonification's potential spans various fields, from scientific research and medical diagnostics to education and accessibility. The development of sophisticated sonification techniques empowers individuals with visual impairments, providing an alternative way to access and interpret information. This demonstrates a powerful inclusionary impact and highlights the versatility of sonification.
One prominent example is the use of sonification in medical diagnostics. Doctors may utilize sonification to analyze heart rhythms or brainwave patterns, providing auditory cues to identify abnormalities. Similarly, scientists can use sonification to analyze seismic data or explore patterns in astronomical observations. These applications demonstrate the power of sonification in improving the efficiency and accuracy of data analysis across various disciplines. Another example comes from the field of education, where sonification can provide students with a novel and engaging way to learn about complex concepts. For instance, visualizing mathematical equations or geographic data through sound can improve understanding and retention.
The effective design of a sonification system requires careful consideration of several factors, including the type of data being represented, the target audience, and the intended purpose. Effective mapping of data to auditory features (pitch, rhythm, timbre, etc.) is critical for creating clear and meaningful sonifications. Poorly designed sonifications can be confusing or even misleading, undermining their utility. A well-designed system, however, offers an additional dimension to data analysis, allowing users to detect patterns and anomalies that might be missed through visual inspection alone. Furthermore, the development of innovative techniques, such as interactive sonification, promises to further enhance the utility and user experience of sonification systems. Interactive sonification allows users to manipulate the sound parameters to explore the data in a more dynamic and engaging way.
The integration of AI and machine learning offers exciting possibilities for automated sonification and the creation of more sophisticated mapping techniques. AI algorithms can analyze large datasets and automatically generate meaningful sonifications, thereby easing the process for researchers and practitioners. Moreover, the use of AI could further personalize sonification experiences by adapting the sound parameters to individual users’ preferences and abilities.
3D Audio in Virtual and Augmented Reality: A Seamless Blend
The integration of 3D audio in virtual and augmented reality (VR/AR) environments significantly enhances immersion and realism. By creating a realistic sense of space and positioning sounds within the virtual world, 3D audio transforms the way users interact with these environments. Imagine walking through a virtual forest and hearing birds chirping from specific locations in the trees, or participating in a virtual conference where voices appear to emanate from the virtual participants. This level of auditory detail creates a more believable and engaging experience.
One compelling example is the use of 3D audio in VR gaming, where realistic sound effects enhance the gameplay experience and improve spatial awareness. Players can more easily locate enemies or react to environmental cues, leading to a more immersive and engaging experience. Another prominent example is the use of 3D audio in virtual tours and simulations. In a virtual museum tour, for example, 3D audio can enhance the experience by placing the sounds of museum artifacts within their respective locations within the virtual space. This creates a more immersive and informative experience for users. Furthermore, the use of 3D audio in VR training simulations can provide a more realistic and effective learning experience by simulating realistic environmental sounds, improving learning outcomes and knowledge retention.
Developing effective 3D audio for VR/AR requires careful consideration of several factors, including the spatial characteristics of the virtual environment, the positioning of sound sources, and the type of headphones or speakers being used. Furthermore, the optimization of 3D audio for different devices and platforms is essential for ensuring a consistent and high-quality listening experience across various user devices. In addition to the technical challenges, there are creative considerations. Sound designers must not only accurately position sounds in the virtual environment, but they must also create a soundscape that complements the visual elements and enhances the overall user experience. Poorly implemented 3D audio can result in a disorienting or even jarring experience, detracting from the overall quality of the virtual environment.
The future of 3D audio in VR/AR is promising, with ongoing advancements in technology leading to more realistic and immersive listening experiences. The integration of AI-powered sound design tools could automate aspects of the 3D audio production process, reducing the workload on sound designers. Furthermore, advancements in haptic feedback technology could provide users with an additional sensory dimension, creating a multi-sensory experience that enhances immersion further.
Personalized Audio Experiences: Tailored to the Individual
Personalized audio experiences are revolutionizing the way we consume sound. By adapting audio settings to individual preferences and auditory characteristics, personalized audio aims to optimize the listening experience and maximize enjoyment. This is particularly important for those with hearing impairments or specific preferences. Imagine a music streaming service that automatically adjusts the equalization to your hearing profile, or a podcast app that optimizes the audio levels to compensate for background noise. Personalized audio offers a much more customized listening experience than traditional approaches.
One significant example is the use of personalized audio in hearing aids, where advanced algorithms adjust the sound settings to compensate for individual hearing loss. Hearing aids can now analyze the listener's auditory profile and automatically adjust volume and equalization settings to optimize the listening experience. Another compelling case study involves the development of personalized music streaming services. These services use machine learning algorithms to analyze user listening history and preferences to suggest music that aligns with individual tastes. This creates a more tailored and enjoyable listening experience, enhancing user satisfaction.
Effective implementation of personalized audio requires collecting and analyzing user data to understand individual auditory preferences and characteristics. This might involve collecting data on hearing loss, preferred listening levels, and musical tastes. Furthermore, the development of robust algorithms and personalized equalization settings are crucial for effective personalization. However, the collection and use of user data must adhere to strict privacy and ethical standards to ensure user trust and protect sensitive information. It's crucial to balance the desire for personalization with respect for user privacy and data security. Poorly implemented personalized audio can result in biased recommendations or inaccurate audio adjustments, compromising user satisfaction and even causing discomfort or inconvenience.
The future of personalized audio involves further advancements in AI and machine learning algorithms that can analyze vast amounts of user data to provide more accurate and sophisticated personalized listening experiences. Advancements in AI could also allow for real-time adjustments to the audio settings, compensating for changing listening environments or user preferences. Moreover, integrating physiological data, such as heart rate and brainwave activity, into the personalization process could further enhance the accuracy and effectiveness of personalized audio systems.
Bioacoustic Monitoring and Environmental Sound Analysis
Bioacoustic monitoring utilizes sound recording and analysis techniques to study animal behavior and monitor environmental changes. By analyzing the sounds produced by animals, researchers can gain insights into their populations, distribution, and behavior. This is particularly valuable for studying elusive species or those inhabiting remote areas. Furthermore, the analysis of environmental sounds can provide insights into broader ecosystem health and identify potential threats, such as pollution or habitat degradation. Bioacoustic monitoring is becoming an increasingly valuable tool for conservation efforts and environmental management.
A noteworthy example of bioacoustic monitoring is its use in studying endangered species. By analyzing the calls of endangered animals, researchers can track their populations, assess their reproductive success, and identify threats to their survival. Another compelling example involves monitoring the impacts of human activity on wildlife. By analyzing the soundscapes of various environments, researchers can assess the noise pollution and evaluate its influence on animal behavior and communication. This provides critical data for informed decision-making related to environmental protection. Additionally, analysis of specific sounds, such as the calls of amphibians, can provide data that is used to create bioacoustic indices of ecosystem health.
Effective bioacoustic monitoring relies on the development of advanced audio recording and analysis techniques. This includes the use of high-quality microphones, specialized recording equipment, and sophisticated software for processing and analyzing large datasets. Furthermore, the use of machine learning and artificial intelligence has greatly improved the ability to automatically identify and classify different animal sounds and other auditory signals, expediting data analysis and providing more accurate results. However, the proper use and interpretation of bioacoustic data require expertise in both audio engineering and ecology. Incorrect interpretation of bioacoustic data can lead to misguided conservation efforts and poor management decisions. Therefore, it's imperative that bioacoustic monitoring projects are conducted by experienced professionals who understand the limitations and potential biases of bioacoustic data.
The future of bioacoustic monitoring is characterized by the ongoing integration of novel technologies and advancements in data analysis methods. The application of advanced machine learning techniques promises to improve the accuracy and efficiency of animal sound identification, leading to more reliable population estimates and improved conservation strategies. Furthermore, the development of more sophisticated sensor networks will allow for broader-scale monitoring of animal populations and environmental conditions, thereby enhancing the resolution and comprehensiveness of bioacoustic data.
Conclusion
The evolution of audio technology is reshaping our interactions with sound. From immersive soundscapes and data sonification to personalized listening experiences and bioacoustic monitoring, the innovations discussed showcase the transformative potential of audio. These advancements are not only enriching our entertainment and communication but also providing valuable tools for scientific research, environmental monitoring, and accessibility. The future of audio promises even greater integration across various sectors, blurring the lines between the digital and physical worlds and offering profound implications for how we interact with information and our environment. Continued research and development in AI, machine learning, and sensory integration will undoubtedly lead to further breakthroughs in audio technology and its applications.