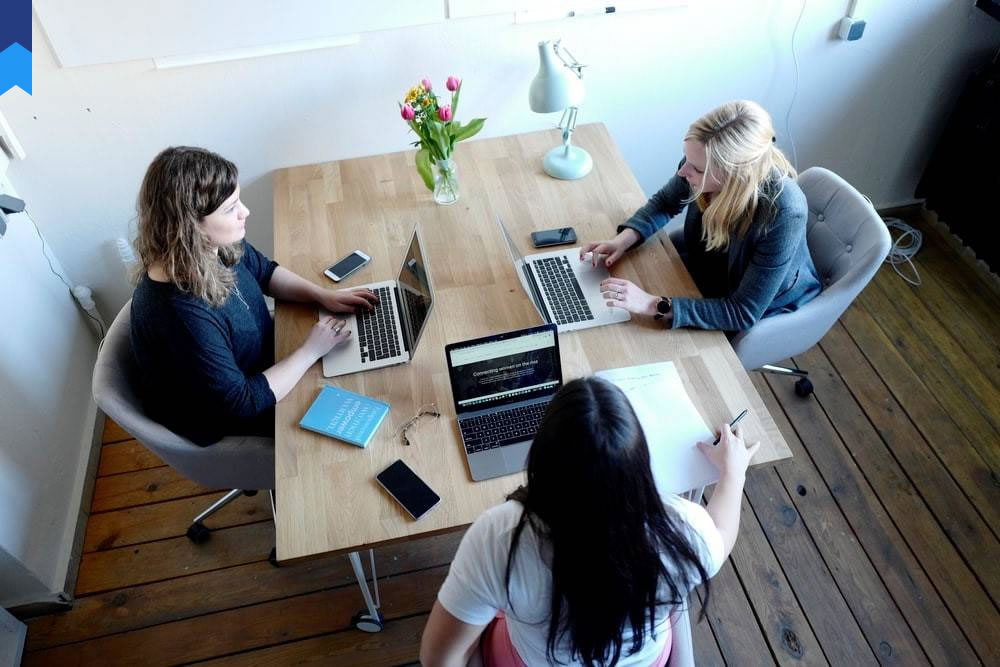
Rethinking Pulse Circuits: A Fresh Perspective On Design And Implementation
Pulse circuits, the foundational building blocks of digital systems, often get overlooked in favor of more complex integrated circuits. However, a deeper dive reveals their enduring relevance and potential for innovation. This article challenges conventional wisdom, offering a fresh perspective on pulse circuit design and implementation, exploring specific, practical, and innovative aspects beyond basic overviews.
Understanding the Fundamentals of Pulse Circuits
Pulse circuits operate based on the principles of digital logic, processing discrete signals rather than analog waveforms. They are characterized by their ability to generate, shape, and manipulate short bursts of electrical energy known as pulses. These pulses serve as the fundamental units of information in digital systems, carrying data and controlling operations. The design of efficient pulse circuits necessitates a thorough understanding of digital logic gates, timing diagrams, and propagation delays. A common example is the use of monostable multivibrators (one-shot timers) for generating precise timing intervals in various applications. One practical case study is the use of pulse circuits in automotive safety systems, where accurate timing is crucial for activating safety features like airbags. In this context, minimizing propagation delays and ensuring reliable pulse generation are essential for optimal performance and safety. Furthermore, considerations like noise immunity and power consumption should be addressed during design, emphasizing the importance of a robust and efficient pulse circuit implementation. The complexity of these systems requires careful attention to detail to ensure smooth operation and reliability. Another case study involves the deployment of advanced pulse shaping techniques in high-speed digital communication systems. To address signal distortion, equalizers are incorporated into pulse circuits. Such equalizers are designed to adjust signal attenuation across the entire spectrum to improve signal integrity and minimize bit errors. The selection of appropriate components, such as transistors, capacitors, and resistors, is paramount in achieving these goals. Proper component selection greatly affects the performance of the circuit and its overall efficiency. Pulse generation methods are diverse; for instance, relaxation oscillators are commonly used due to their simplicity and ease of implementation. Designing for reliability involves incorporating error detection and correction schemes, which require additional circuit components and complexity, leading to trade-offs between performance, reliability, and cost. This is true of digital circuits in general, and pulse circuits are no exception. The impact of temperature variation on circuit performance also needs consideration. Temperature-stable components should be chosen and appropriate compensation techniques implemented to ensure the reliability of the circuit under changing environmental conditions.
Innovative Approaches to Pulse Circuit Design
Beyond traditional methodologies, innovative approaches are transforming pulse circuit design. One prominent area is the exploration of integrated circuit technology to create highly integrated, low-power pulse generators. Modern CMOS (Complementary Metal-Oxide-Semiconductor) technology enables the creation of compact, energy-efficient pulse circuits. The use of advanced fabrication techniques leads to smaller devices, requiring lower power and producing less heat. This is crucial for battery-powered applications. For instance, many portable devices rely on these energy-efficient integrated circuits. A case study involves the design of microcontrollers that utilize sophisticated pulse-width modulation (PWM) techniques for precise control of motors and other actuators. These advancements have enabled the development of sophisticated and miniature electronic devices. Another case study focuses on the utilization of field-programmable gate arrays (FPGAs) for rapid prototyping and customization of pulse circuits. FPGAs offer flexibility for testing different designs before committing to a physical implementation. They provide a faster and more cost-effective approach to circuit development, reducing time-to-market. A common use of FPGAs includes the testing of complex digital signal processing algorithms in real-time, prior to integration into a final system. This is very common in modern signal processing applications that utilize sophisticated algorithms to perform tasks ranging from noise reduction to signal encryption. The rapid pace of advances in both FPGA technology and pulse circuit design is leading to further innovation in this field. The use of artificial intelligence and machine learning algorithms is a very recent advancement in pulse circuit design. These techniques can improve system performance by adapting and optimizing pulse parameters in real-time, based on the specific application demands. Machine learning is being used to predict and mitigate anomalies, thereby improving efficiency, safety, and reliability of pulse circuits. The development of more complex functionalities and higher levels of integration would lead to further increase in efficiency and a reduction in overall size and cost. Further developments are also required for improved thermal management, noise reduction, and higher levels of integration.
Advanced Pulse Shaping Techniques for Improved Performance
Pulse shaping plays a crucial role in optimizing the performance of pulse circuits. Techniques such as Gaussian pulse shaping and raised-cosine filtering are used to minimize intersymbol interference (ISI) in high-speed digital communication systems. Gaussian pulse shaping produces pulses with minimal time spread, thereby leading to an improvement in the transmission speed. However, it necessitates more advanced signal processing techniques. Raised-cosine filtering offers a trade-off between pulse spread and filter complexity. One case study highlights the impact of pulse shaping on data transmission rates in high-speed fiber optic communication systems. By employing optimal pulse shaping techniques, significant improvements in bandwidth efficiency have been achieved. This has a profound impact on the capabilities of long-distance communication. Another example includes the use of advanced pulse shaping in radar systems to enhance target detection and resolution. Pulse compression techniques are used to achieve high resolution in radar signal processing. The proper selection of the pulse shape determines the overall performance of the radar system. Proper signal shaping can improve the signal-to-noise ratio (SNR), enabling better detection of weak signals in noisy environments. This is especially crucial in military and environmental monitoring applications. Modern radar systems are based on sophisticated algorithms that analyze the returned signals, and appropriate pulse shaping is essential for the proper operation of these algorithms. Future trends in pulse shaping will likely focus on adaptive techniques, where the pulse shape is dynamically adjusted to optimize performance in varying conditions. This will necessitate the development of sophisticated signal processing algorithms to enable such adaptive pulse shaping capabilities. Moreover, integration of AI and machine learning is expected to play a significant role in the automatic optimization and adjustment of pulse shapes, leading to significant improvements in the efficiency and performance of many applications.
Practical Applications and Case Studies
Pulse circuits find widespread applications across various fields. In medical imaging, precise pulse generation is essential for accurate image acquisition in techniques such as Magnetic Resonance Imaging (MRI). MRI pulse sequences require very precise pulse generation and control to achieve high-quality images of internal organs. A case study involves the design of highly specialized pulse generators for MRI machines, which need to meet very stringent requirements in terms of accuracy and stability. Another application is in industrial automation, where pulse circuits are used to control the operation of machines and robotics. Precise timing is essential in automated assembly lines, and any error can lead to malfunctions. A case study focuses on the use of pulse circuits in robotics for fine motor control, where the precise timing of signals is essential for accurate movements. Pulse circuits are also crucial in power electronics, where they are used to control switching devices in converters and inverters. In high-voltage power supplies, pulse circuits play a crucial role in regulating the flow of power. A case study demonstrates the use of pulse-width modulation (PWM) techniques in power converters to efficiently manage the flow of electrical energy. This technology significantly improves power efficiency and reduces energy waste. Another case study analyzes the applications of pulse circuits in modern aerospace engineering, where they are employed in various control systems and communication networks. This shows the criticality of reliable and highly performant pulse circuits in these sophisticated applications. The future implications of pulse circuit technology are promising, given the continuing advancements in semiconductor technology and the rising demand for faster, more efficient electronic systems. Further research and development efforts in pulse circuit design are essential for advancing technology in these various application areas. This is true for medical applications, where higher precision is constantly required, and industrial automation, where higher speed and accuracy is constantly sought after.
Challenges and Future Directions
Despite their importance, pulse circuit design faces challenges. Minimizing power consumption remains a significant area of focus, particularly in portable and embedded systems. The development of novel circuit architectures and low-power components is crucial for addressing this challenge. Another significant hurdle is reducing electromagnetic interference (EMI), which can affect the reliability and performance of pulse circuits. The implementation of appropriate shielding and filtering techniques are essential for mitigating EMI effects. A key future direction involves the integration of advanced materials and nanotechnology to create more efficient and compact pulse circuits. This is essential for creating smaller and more powerful circuits in the future. Moreover, the exploration of novel circuit architectures and design methodologies, such as the use of memristors and other emerging technologies, holds immense promise for improved efficiency and performance. Another challenge is the testing and verification of complex pulse circuits. The development of advanced simulation and verification tools is essential for ensuring the reliability and correctness of pulse circuit designs. Further research is needed to explore the limitations and potential of the various pulse circuit designs that have been proposed. This would include studying their potential shortcomings and areas where improvements are needed. This will necessitate a combination of theoretical analysis, experimental verification, and the development of new design methods. The future of pulse circuit design is tied to the advancement of broader fields in electronics, including integrated circuit technology and advanced materials research. The development of these fundamental technologies will directly impact and facilitate the development of advanced pulse circuits for the future. Exploring and implementing advanced error correction techniques is vital for improving the reliability of pulse circuits in harsh operating environments. This becomes especially important in applications such as aerospace and deep-sea exploration. These applications demand extremely reliable systems.
Conclusion
Pulse circuits, despite their seemingly simple nature, are fundamental to modern electronics. This article has challenged conventional views, offering a fresh perspective on design and implementation. By exploring innovative approaches, advanced shaping techniques, and real-world applications, we have highlighted the ongoing relevance and future potential of pulse circuits. Addressing the challenges of power consumption, EMI, and testing will be vital for pushing the boundaries of this critical technology. The continued integration of AI, nanotechnology, and advanced materials science will shape the future of pulse circuits, driving innovation and enabling groundbreaking advancements across numerous fields. The ongoing research and development in this area promise to bring about further improvements in both performance and efficiency.