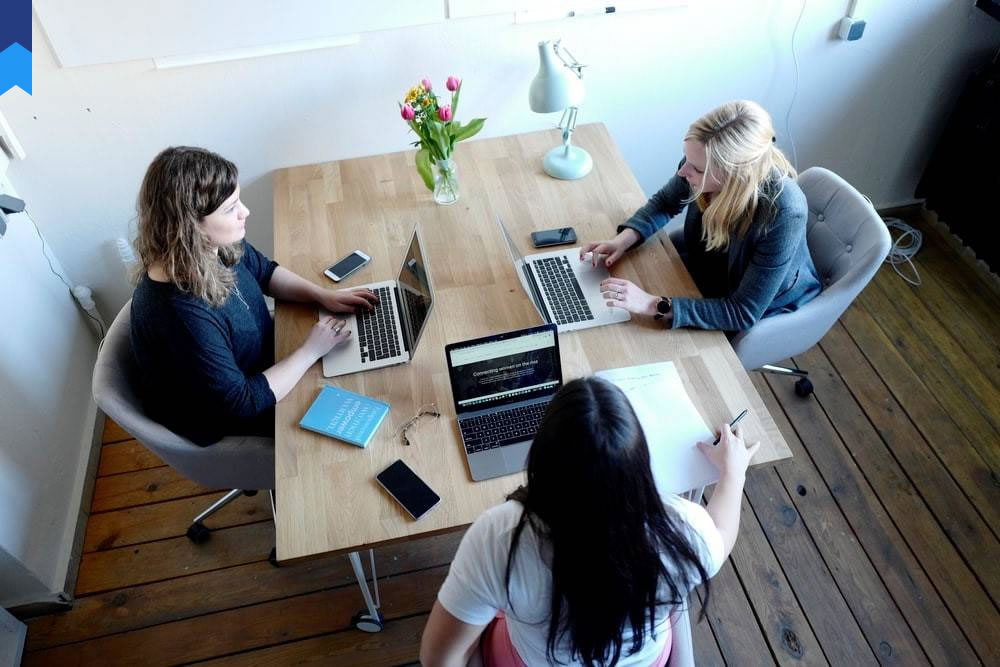
The Hidden Mechanics Of Solar Power Optimization
Harnessing the sun's energy efficiently is more than just installing panels. This article delves into the often-overlooked complexities of optimizing solar energy systems, revealing the hidden mechanics that significantly impact performance and return on investment.
Optimizing Panel Placement and Angle
The sun's path across the sky varies dramatically throughout the year. Optimizing panel placement requires careful consideration of this variation to maximize energy capture. A south-facing installation (in the Northern Hemisphere) is generally ideal, but even a slight deviation can lead to noticeable losses. The optimal tilt angle depends on latitude and the goal of either maximizing winter or summer production. For example, a steeper angle works best in winter to catch low-angle sunlight, while a shallower angle is better for summer.
Case Study 1: A residential installation in a high-latitude region experienced a 15% increase in annual energy production by adjusting panel tilt based on seasonal changes. This involved using a motorized tracking system to dynamically adjust panel angles.
Case Study 2: A commercial solar farm in a low-latitude area with extensive shading saw improved energy generation by employing sophisticated panel placement software that calculated optimal positions while minimizing shade impact. This involved detailed 3D modeling of the site and the sun's path to simulate various scenarios and pinpoint the ideal positioning.
Software tools like PVsyst and Helioscope are increasingly used to simulate different panel orientations and determine the most efficient configuration for specific locations. These tools also account for factors such as shading from trees, buildings, and other structures. Industry best practice recommends a site survey to assess shading conditions and other environmental variables before finalizing panel placement.
The precise angle of incidence of sunlight on the panel is crucial. The best angle is perpendicular to the sun’s rays, maximizing the light absorbed. This dynamic change is the principle behind dual-axis solar trackers, which rotate to follow the sun's movement, thereby constantly optimizing this angle throughout the day. However, these systems are more complex and expensive than fixed-tilt systems. The choice between a fixed-tilt or tracking system involves a cost-benefit analysis considering the increase in energy production versus the higher initial investment.
A well-planned layout minimizes shading effects between panels. This careful arrangement, often facilitated by computer modeling, is paramount for maximizing energy output. Overcrowding panels, for example, can cause significant losses as panels shade each other.
Advanced techniques include using bifacial panels, which capture light from both sides. These require more careful placement to maximize back-reflection, but they can boost energy production by up to 30%. The potential gains must be weighed against the higher cost of bifacial panels and the need for optimized ground reflectance.
Proper installation techniques are vital for optimal performance. Panels must be securely mounted and electrically connected without gaps or poor wiring that could lead to voltage loss. Regular inspections and maintenance are crucial to maintaining optimal energy production.
Microinverters and Optimizers: Maximizing Energy Harvest
Traditional string inverters convert the DC power from a series of panels into AC power. If one panel in the string is shaded or malfunctioning, it affects the entire string's output. Microinverters, on the other hand, convert the DC power from each individual panel. This allows for independent optimization, meaning a single faulty or shaded panel won't impact the rest. This approach provides greater resilience and efficiency compared to string inverters, which is crucial for maximizing output in varied weather conditions.
Case Study 1: A large-scale solar installation using microinverters experienced a 10% improvement in energy yield compared to a similar system using string inverters in a region prone to partial shading from cloud cover. The individual optimization offered by microinverters provided a significant increase in overall efficiency.
Case Study 2: A residential solar system with a complex roof configuration benefited greatly from the use of microinverters. Partial shading caused by chimneys and vents would have severely hampered the performance of a string inverter system, but the microinverters ensured maximum energy production from each panel, regardless of shading.
Power optimizers offer a middle ground between string inverters and microinverters. They're placed between each panel and the string inverter, allowing for individual monitoring and voltage optimization. This allows for improved power collection, even under less-than-ideal conditions. Though not as independently effective as microinverters, they represent a good balance between cost and performance.
The choice between microinverters, power optimizers, and string inverters involves weighing energy yield, cost, and complexity. A detailed analysis of site-specific conditions is needed for making an optimal choice.
Monitoring systems play a key role in optimizing energy harvest. They track panel performance, identifying potential issues like shading, soiling, or malfunctions. Real-time data helps in proactive maintenance and keeps the system running at peak efficiency.
Advanced algorithms and AI are now being incorporated into monitoring systems to predict and prevent potential problems before they impact energy production. This data-driven approach optimizes performance and minimizes downtime.
Regular cleaning of panels is essential. Dust, bird droppings, and other debris can significantly reduce energy generation. A clean panel surface is crucial for maintaining peak performance.
Battery Storage and Energy Management
Battery storage systems allow for storing excess solar energy generated during peak production for use during periods of low production, like nighttime or cloudy days. This increases self-consumption and reduces reliance on the grid. This capability is crucial for increasing the overall return on investment in the solar system.
Case Study 1: A household equipped with a battery storage system experienced a 75% reduction in their reliance on grid electricity after installing their solar panels. This significant reduction led to substantial cost savings.
Case Study 2: A commercial business using battery storage for backup power was able to continue operations seamlessly during a grid outage. The uninterrupted power supply protected critical systems and minimized financial losses.
Battery technology is constantly evolving. Lithium-ion batteries are currently the most common choice, but other chemistries like solid-state batteries show promise for higher energy density and improved safety. Battery management systems are crucial for monitoring and optimizing battery performance, maximizing lifespan and safety.
Intelligent energy management systems use algorithms to optimize energy flow between the solar panels, batteries, and grid. They prioritize self-consumption and maximize the use of stored energy before drawing power from the grid. Advanced systems can even integrate with smart home technology, allowing for automated control of energy use.
The cost of battery storage remains a significant factor. The upfront cost of batteries needs to be balanced against the long-term savings from reduced grid electricity consumption. Government incentives and rebates can help make battery storage more affordable.
Battery lifespan is crucial. Proper maintenance and temperature control can prolong battery life and ensure a good return on investment. Choosing a high-quality battery system with a strong warranty is essential.
The integration of battery storage into solar energy systems marks a significant step towards energy independence and grid stability. It is a crucial component of a robust and efficient solar energy solution. Future developments in battery technology promise to further improve cost-effectiveness and performance.
Grid Integration and Net Metering
Efficient integration of solar energy systems into the grid is vital for both the home and utility companies. Net metering policies, in place in many regions, allow for credits on electricity bills for excess energy fed back into the grid. This can significantly reduce or even eliminate electricity bills. Understanding the regulations and the technical aspects of grid interaction is essential for maximizing the financial benefits of a solar system.
Case Study 1: A homeowner participating in a net metering program received substantial credits on their electricity bills, effectively offsetting their energy consumption. This showed the significant financial advantages of net metering.
Case Study 2: A community solar farm that successfully integrated into the local grid helped reduce the overall carbon footprint of the region. This highlighted the positive contribution of community solar initiatives.
Grid inverters are key components of grid integration. They convert DC power from the solar panels into AC power that is compatible with the grid. These inverters must meet specific safety and performance standards to ensure grid stability.
The effectiveness of net metering can vary depending on local regulations. Understanding the specific terms and conditions of a net metering program is crucial for making informed decisions. These rules often impact factors such as the rate of credit given for excess energy and any potential limitations on the amount of energy exported to the grid.
Anticipating future changes in net metering policies is crucial. Government regulations evolve, so staying informed about any changes can help avoid future unexpected financial impacts.
Modern smart grid technologies are improving grid integration efficiency. These technologies enhance communication between the solar system and the utility grid, allowing for better load balancing and grid stability. These advancements in technology help optimize the usage of solar energy and improve grid management.
Understanding the grid's capacity is crucial before installing a large-scale solar system. Excessive energy production might overwhelm the local grid infrastructure, and the proper planning and cooperation with the utility company are essential to avoid problems.
Monitoring, Maintenance, and Long-Term Optimization
Continuous monitoring of a solar energy system's performance is crucial for maximizing its lifespan and efficiency. Regular maintenance helps prevent problems before they significantly impact production. This proactive approach is key to maintaining optimal performance and long-term energy generation.
Case Study 1: A systematic maintenance plan resulted in a 5% increase in energy production over a five-year period, showcasing the importance of regular maintenance.
Case Study 2: Early detection of a faulty inverter through regular monitoring prevented a complete system failure and significant energy losses, demonstrating the value of preventative maintenance.
Monitoring systems provide real-time data on various parameters, including panel performance, inverter efficiency, and energy consumption. This data helps identify potential issues and allows for timely interventions, preventing major problems.
Regular cleaning of solar panels is vital. Accumulated dust, dirt, and other debris can significantly reduce energy production. A consistent cleaning schedule can maintain optimal performance levels. The frequency of cleaning depends on environmental factors like weather patterns.
Inspections should be conducted at least annually, checking for any damage to panels, wiring, or other components. Early detection and repair of minor issues can prevent costly repairs later on.
Replacing faulty components promptly is essential. Delaying repairs can lead to cascading failures, reducing efficiency and increasing repair costs. A readily available supply of replacement parts is recommended.
Long-term optimization strategies should consider factors like changing environmental conditions and technological advancements. Upgrades or modifications to the system might be necessary over time.
The use of AI-powered predictive maintenance tools helps identify potential issues before they arise. This proactive approach maximizes efficiency and minimizes downtime.
Conclusion: Optimizing a solar energy system is a multifaceted process that goes beyond basic installation. By understanding the hidden mechanics discussed in this article – from optimal panel placement to advanced monitoring and maintenance strategies – homeowners and businesses can significantly improve the efficiency and longevity of their solar investments, ultimately maximizing energy production and reducing their reliance on traditional power sources. Embracing innovative technologies and proactive maintenance is crucial for unlocking the full potential of solar energy and securing a cleaner, more sustainable energy future.