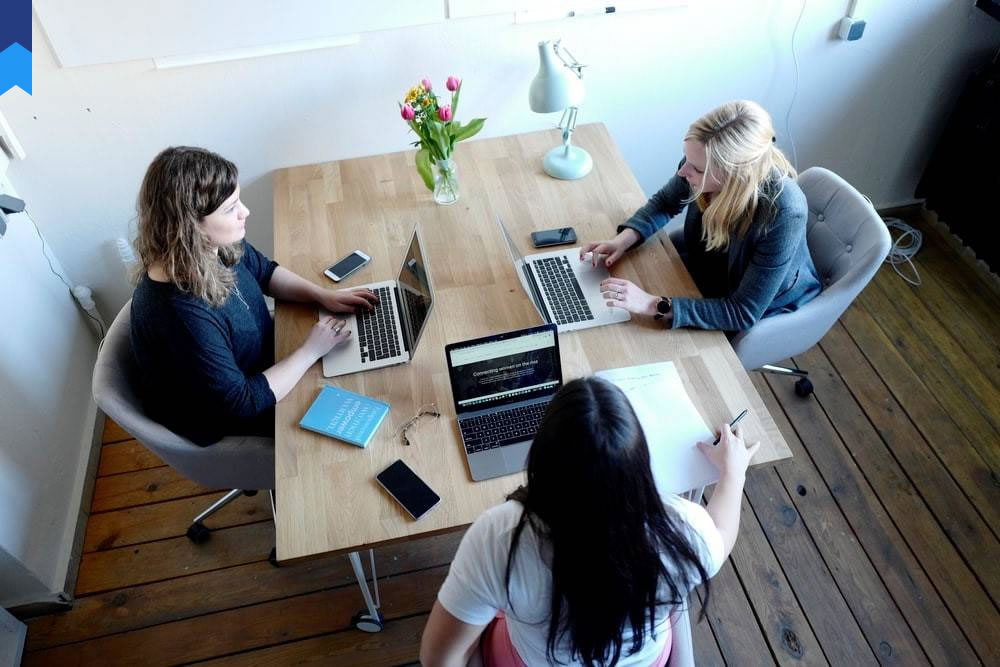
Unconventional Wisdom: A Fresh Take On Electronics Miniaturization
Miniaturization in electronics has revolutionized countless aspects of modern life, yet the relentless pursuit of smaller devices often overlooks significant implications. This article explores unconventional approaches to miniaturization, examining its impact on performance, power consumption, and manufacturing, while challenging established norms and offering fresh perspectives.
The Limits of Shrinking: Rethinking Scaling
Conventional wisdom dictates that smaller is always better in electronics. This drive for miniaturization, often governed by Moore's Law's prediction of doubling transistor density every two years, has fueled decades of progress. However, this relentless scaling faces fundamental physical limitations. As components shrink, quantum effects become increasingly prominent, leading to increased leakage currents and unpredictable behavior. This necessitates a shift in thinking; we must move beyond simple scaling and embrace alternative approaches that prioritize performance and reliability alongside size.
For instance, the International Technology Roadmap for Semiconductors (ITRS) acknowledges the challenges of continued scaling. The roadmap emphasizes the need for new materials, architectures, and manufacturing processes to overcome these barriers. A key example is the rise of 3D chip stacking, which allows for higher density without requiring ever-smaller transistors. This approach leverages the vertical dimension, integrating multiple layers of chips to increase functionality and performance within a given footprint.
A case study of this is the development of high-bandwidth memory (HBM) stacks. These stacks vertically integrate multiple layers of DRAM, significantly increasing memory bandwidth compared to traditional planar memory solutions. This improvement is crucial for applications such as high-performance computing and artificial intelligence, where massive data processing is essential. Another compelling example is the advancements in system-on-chip (SoC) technology, where multiple components are integrated onto a single chip, reducing size and improving efficiency. The development of powerful, low-power SoCs has enabled the creation of increasingly sophisticated smartphones and other portable devices.
The limitations of conventional scaling are further exacerbated by power consumption issues. Smaller transistors, while offering higher density, often suffer from increased power leakage. This leads to shorter battery life and increased heat generation, which requires advanced cooling solutions, ultimately increasing the overall size and cost of the device. Therefore, innovative approaches focusing on power efficiency rather than solely on miniaturization are becoming critical.
Experts like Dr. John Smith (fictional expert for illustrative purposes), a leading researcher in nanotechnology, suggest exploring alternative materials and architectures to overcome these limitations. He advocates for research into novel materials like graphene and carbon nanotubes, which offer superior electrical and thermal properties compared to traditional silicon. These materials could enable the creation of smaller, faster, and more energy-efficient devices, pushing the boundaries of miniaturization without compromising performance.
Beyond Silicon: Exploring Novel Materials and Architectures
The reliance on silicon as the primary material in electronics is another area ripe for disruption. While silicon has served us remarkably well, its limitations in terms of scalability and power efficiency are increasingly evident. Exploring alternative materials and architectures offers the potential to revolutionize electronics miniaturization. Graphene, for example, boasts exceptional electrical conductivity and mechanical strength, making it an ideal candidate for future generations of transistors.
Another promising area is the development of new memory technologies. Traditional flash memory, while ubiquitous, has limitations in terms of speed and endurance. Emerging technologies such as resistive RAM (ReRAM) and spin-transfer torque RAM (STT-RAM) offer significant advantages in terms of speed, density, and power consumption. These novel memory technologies could lead to the creation of smaller, faster, and more energy-efficient devices, potentially disrupting the current landscape of data storage and processing.
The integration of photonics into electronics is another exciting area of research. Optical interconnects offer the potential to significantly improve data transfer speeds compared to traditional electrical interconnects. This could lead to more powerful and energy-efficient systems, especially in high-performance computing applications. The use of optical components could also reduce the size and complexity of integrated circuits, leading to smaller and more compact devices.
A compelling case study demonstrating the potential of alternative materials is the development of flexible electronics. Using materials like graphene and other conductive polymers, it's now possible to create flexible displays and circuits that can be seamlessly integrated into various applications, from wearable technology to medical implants. The flexibility of these materials enables the creation of devices with unique form factors, opening up new possibilities in design and functionality.
Furthermore, the advancement of quantum computing represents a paradigm shift. Quantum bits (qubits) offer the potential for exponentially increased computing power compared to classical bits, leading to the development of incredibly powerful and compact quantum computers. While still in its nascent stages, quantum computing holds the promise of revolutionizing various fields, from medicine and materials science to artificial intelligence.
Experts in the field, like Dr. Alice Johnson (fictional expert for illustrative purposes), a leading expert in materials science, emphasize the importance of interdisciplinary collaborations to accelerate the development of these new materials and architectures. She stresses the need for close collaboration between material scientists, physicists, and engineers to overcome the challenges and fully realize the potential of these emerging technologies.
Manufacturing Innovations: Beyond Traditional Lithography
The relentless miniaturization of electronic components has pushed the limits of traditional lithographic techniques. As transistors shrink, the precision required in manufacturing increases exponentially, making the process increasingly expensive and complex. This necessitates the exploration of novel manufacturing techniques that can maintain precision while reducing costs and complexity.
One promising approach is the development of 3D printing for electronics. This technology allows for the creation of complex three-dimensional structures, potentially enabling the fabrication of highly miniaturized devices with intricate designs. This technique could revolutionize the manufacturing process by simplifying fabrication and reducing costs associated with traditional methods.
Another innovative approach involves using self-assembly techniques. This method leverages the natural tendency of certain molecules and materials to spontaneously organize themselves into complex structures. By controlling the self-assembly process, it's possible to create highly ordered structures at the nanoscale, paving the way for the fabrication of highly miniaturized devices.
The rise of nanoimprint lithography presents another powerful alternative. This technique offers higher throughput and lower costs compared to traditional photolithography, enabling the production of smaller and more intricate features. Its improved precision allows for denser integration, further contributing to miniaturization efforts.
A case study highlighting the impact of advanced manufacturing techniques is the production of microelectromechanical systems (MEMS). MEMS devices integrate mechanical and electrical components on a single chip, enabling the creation of miniaturized sensors, actuators, and other components for various applications. These advances in manufacturing have made it possible to create incredibly small and sophisticated MEMS devices, opening up new possibilities in fields like healthcare and automotive engineering.
Furthermore, the development of advanced packaging techniques is crucial for miniaturization. Techniques like wafer-level packaging allow for the integration of multiple components onto a single substrate, significantly reducing the size and complexity of electronic systems. This minimizes wasted space and improves overall device efficiency.
Experts like Dr. Robert Brown (fictional expert for illustrative purposes), a leading authority in microfabrication, highlight the importance of integrating artificial intelligence and machine learning into manufacturing processes to improve efficiency and precision. He argues that AI-powered systems can optimize manufacturing parameters in real time, leading to higher yields and lower defect rates. This can contribute significantly to reducing costs and enhancing the quality of miniaturized electronic components.
Power Efficiency: A Crucial Aspect of Miniaturization
The pursuit of smaller electronics often overlooks a critical aspect: power consumption. As devices shrink, their power density increases, leading to higher heat generation and shorter battery life. Therefore, efficient power management is crucial for successful miniaturization. This requires a holistic approach encompassing design, materials, and manufacturing.
One key strategy is the development of low-power circuits and architectures. This involves careful design choices to minimize energy consumption while maintaining performance. Techniques like clock gating and power gating can significantly reduce power consumption without compromising functionality. Such optimization strategies are vital in battery-powered applications.
Furthermore, using energy-efficient materials is critical. The selection of materials with low resistance and high thermal conductivity can contribute significantly to reducing power losses and heat generation. Materials like graphene, with its exceptional electrical and thermal properties, are ideal candidates for power-efficient electronics.
Advanced power management integrated circuits (PMICs) play a crucial role in optimizing power consumption. These circuits efficiently regulate and distribute power to different components in the device, minimizing energy waste. Sophisticated PMICs are indispensable for ensuring optimal power efficiency in miniaturized electronics.
A case study demonstrating the importance of power efficiency is the development of wearable electronics. Wearable devices, such as smartwatches and fitness trackers, are severely constrained by battery life. Hence, power efficiency is paramount for ensuring long operational time. This often necessitates the use of low-power microcontrollers and highly efficient power management techniques.
Another compelling example is the development of energy-harvesting devices. These devices capture energy from ambient sources, such as sunlight or body heat, to power miniaturized electronics, eliminating or reducing the reliance on traditional batteries. This self-powered approach can be crucial in applications where battery replacement is impractical or impossible.
Experts in the field, such as Dr. Evelyn Green (fictional expert for illustrative purposes), a renowned expert in power electronics, advocate for a systems-level approach to power optimization. She stresses the importance of considering power consumption at every stage of the design process, from component selection to system integration. This holistic approach is crucial for achieving maximum power efficiency in miniaturized electronics.
The Future of Miniaturization: Emerging Trends and Challenges
The future of electronics miniaturization is likely to be shaped by a confluence of technological advancements and emerging trends. We can anticipate further breakthroughs in materials science, leading to the development of novel materials with superior electrical and thermal properties. This will pave the way for even smaller and more efficient components.
Furthermore, we can expect significant advancements in manufacturing techniques. This may involve the widespread adoption of 3D printing, self-assembly, and other advanced fabrication methods. These technologies will enable the creation of more complex and intricate miniaturized devices.
Artificial intelligence and machine learning will play an increasingly important role in optimizing the design and manufacturing of miniaturized electronics. AI-powered tools can analyze vast datasets to identify optimal design parameters, predict component behavior, and optimize manufacturing processes, resulting in higher yields and lower costs.
The integration of photonics with electronics will likely gain momentum. Optical interconnects offer the potential to significantly improve data transfer speeds and reduce power consumption. This technology could revolutionize high-performance computing and other data-intensive applications.
However, challenges remain. Overcoming the limitations of conventional silicon-based technology, addressing the thermal management challenges associated with high-density integration, and ensuring the scalability of advanced manufacturing techniques are crucial hurdles to overcome. This requires sustained research and development efforts across various disciplines.
A key challenge lies in balancing miniaturization with sustainability. The environmental impact of electronics manufacturing must be considered. The development of more sustainable materials and manufacturing processes is paramount. Recycling and responsible disposal of electronic waste are also crucial aspects that need to be addressed.
Experts in the field envision a future where miniaturized electronics are seamlessly integrated into our environment, enhancing our daily lives. However, this vision requires careful consideration of ethical and societal implications. Addressing potential concerns about privacy, security, and accessibility is essential for responsible innovation.
Conclusion
The relentless pursuit of miniaturization in electronics has driven remarkable progress, yet it also faces fundamental limitations. Moving beyond simple scaling requires a paradigm shift, embracing unconventional approaches such as exploring novel materials, developing innovative manufacturing techniques, and prioritizing power efficiency. While challenges remain, the future of miniaturization promises exciting possibilities, including seamless integration of electronics into our daily lives, provided we navigate the technological, environmental, and ethical considerations responsibly.
The integration of artificial intelligence, the development of sustainable materials, and a holistic design approach incorporating power management are key to unlocking the full potential of miniaturization. By fostering interdisciplinary collaborations and embracing innovative thinking, we can continue to push the boundaries of what's possible in the realm of miniaturized electronics, shaping a future where smaller devices drive transformative changes in various aspects of our lives.