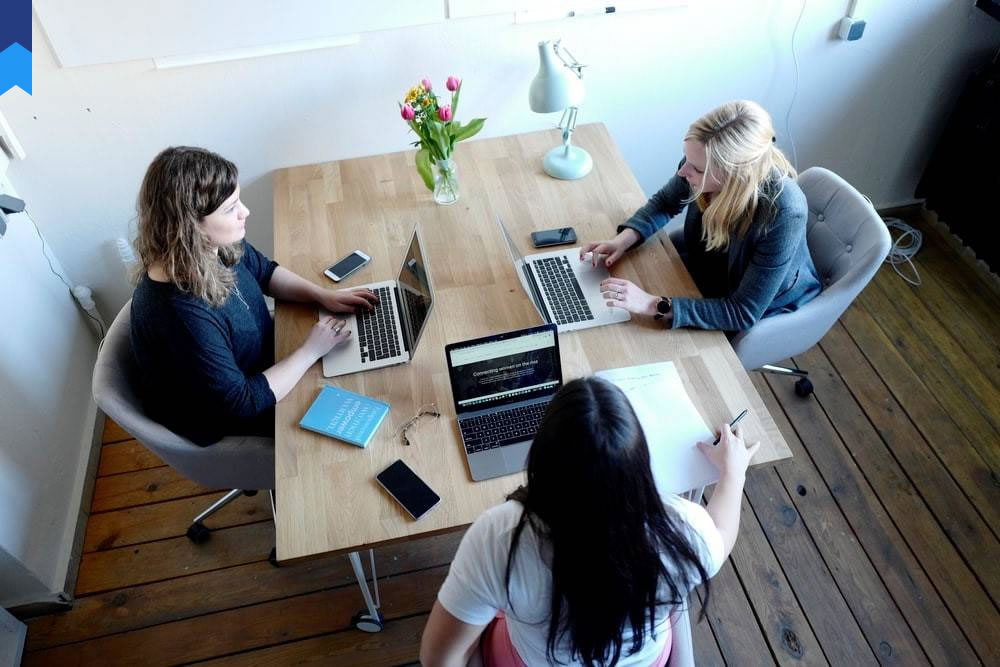
Hidden Truths About Concrete: Mastering Modern Mix Designs
Concrete, the ubiquitous building material, often hides surprising complexities. While seemingly simple, its properties and applications are far richer than most realize. This article delves into the hidden truths behind concrete engineering and technology, revealing innovative techniques and unexpected challenges in modern mix design.
Section 1: The Alchemy of Aggregates: Unveiling the Unsung Heroes
Aggregates, the foundational components of concrete, significantly impact its overall performance. The selection of aggregates is far from arbitrary; factors like particle size distribution, shape, and mineralogy subtly yet profoundly influence strength, durability, and workability. Poor aggregate selection can lead to significant issues like segregation, reduced strength, and increased susceptibility to cracking.
For instance, using excessively angular aggregates can lead to higher water demand during mixing, decreasing the ultimate compressive strength. Conversely, rounded aggregates can improve workability but may compromise long-term strength. A case study in California highway construction highlighted the importance of meticulous aggregate selection. The use of locally sourced aggregates, initially deemed cost-effective, ultimately resulted in significant cracking and premature failure due to their high alkali-aggregate reactivity. This costly mistake underscored the need for thorough testing and analysis. Another case study from a high-rise construction project in Dubai showcased the advantages of optimizing aggregate blending. By carefully selecting a mix of different sized and shaped aggregates, engineers were able to reduce the required water content significantly while achieving enhanced durability. This careful consideration minimized shrinkage and cracking and ensured the structural integrity of the building.
Optimal aggregate gradation is crucial. A well-graded mix minimizes voids, resulting in denser, stronger concrete. Conversely, poorly graded mixes can exhibit increased porosity, making them more susceptible to damage from freeze-thaw cycles or chemical attack. Moreover, the type of aggregate influences concrete's response to environmental factors. For example, reactive aggregates can interact with alkalis in cement, leading to expansion and cracking over time. Extensive laboratory testing and detailed geological surveys are essential to avoid these potential pitfalls. The understanding of aggregate properties, including their mineralogy and reactivity, is crucial to creating durable and high-performing concrete structures.
Understanding the nuances of aggregate selection and gradation is paramount in achieving optimal concrete performance. This goes beyond simply choosing the cheapest option; it involves a sophisticated understanding of material science and a commitment to rigorous testing. This careful consideration not only safeguards the structural integrity of projects but also contributes to increased longevity, cost-effectiveness, and sustainability in concrete construction. This careful approach significantly reduces risks associated with premature failure and contributes to long-term durability and structural integrity.
Section 2: Beyond Compressive Strength: Exploring Concrete's Multifaceted Nature
Concrete's reputation rests largely on its compressive strength. However, this is just one facet of its complex mechanical behavior. Tensile strength, flexural strength, and shear strength are equally crucial for structural integrity, particularly in reinforced concrete structures. Ignoring these properties can lead to structural weaknesses and potential failures.
A significant development is the use of high-performance concrete (HPC), which boasts improved compressive and tensile strength, along with enhanced durability. HPC formulations often incorporate supplementary cementitious materials (SCMs) like fly ash or silica fume, which refine the pore structure and improve the overall performance. A case study of a bridge construction project in Japan highlighted the benefits of HPC. Utilizing HPC allowed for a significant reduction in the structural members' size and weight, leading to cost savings while meeting the stringent performance requirements. The integration of fiber reinforcement, such as steel or synthetic fibers, further enhances the tensile and flexural strength of concrete, creating highly durable composite materials. These fibers improve the toughness and resistance to cracking, improving the overall performance and longevity of the structure.
Another example in high-rise construction in Singapore showed the advantages of using high-strength concrete with fiber reinforcement. The resulting concrete exhibited superior ductility and crack control, mitigating the risks of seismic damage. This strategic approach reduced overall material requirements, enhancing both performance and cost-efficiency. However, the increased cost of HPC and fiber reinforcement needs to be carefully balanced against its potential benefits. Furthermore, the use of HPC requires a more refined understanding of its rheological properties and placement techniques to ensure proper consolidation and minimize the risk of segregation. These enhancements and complexities present new challenges in mix design and implementation. The utilization of advanced simulation and modeling techniques has become imperative for assessing the performance of these enhanced concrete composites.
Understanding the full spectrum of concrete's mechanical properties is critical for designing safe and durable structures. Focusing solely on compressive strength overlooks crucial aspects like tensile and flexural strength, particularly relevant in earthquake-prone regions or in structures subject to dynamic loading. Careful consideration of all relevant mechanical properties ensures optimal structural design and safety.
Section 3: Sustainable Concrete: Reducing the Environmental Footprint
The concrete industry's environmental impact is substantial, primarily due to cement production's high carbon dioxide emissions. This has spurred research and development into sustainable concrete alternatives and practices. The reduction of cement content through the use of SCMs, such as fly ash, slag, and silica fume, is a key strategy. These SCMs not only reduce emissions but also can improve concrete's durability and workability.
The use of recycled materials, including recycled aggregates and industrial byproducts, has gained momentum. A notable case study in Europe demonstrated the feasibility of using recycled concrete aggregates in road construction, showcasing its comparable performance to conventional aggregates while significantly reducing the environmental impact. Moreover, the incorporation of recycled plastics or industrial waste materials offers a further reduction in the need for virgin resources and reduces landfill waste. This innovative approach transforms waste into valuable resources, contributing to a circular economy model in the construction industry.
Another project focusing on green concrete examined the performance characteristics of concrete incorporating fly ash and ground granulated blast-furnace slag (GGBFS). The study indicated improved durability and reduced carbon footprint compared to conventional concrete. Furthermore, the optimization of mix designs through advanced computational modeling helps minimize cement consumption while maintaining the required mechanical properties. The use of self-compacting concrete (SCC) reduces energy consumption during placement and consolidation, further contributing to sustainable construction practices. This approach reduces the need for excessive vibration, minimizing noise pollution and promoting environmentally conscious construction.
Addressing the environmental impact of concrete is paramount for sustainable development in the construction industry. Innovative solutions, such as employing SCMs, recycled materials, and optimizing mix designs, offer a pathway towards a greener concrete future. These efforts play a crucial role in mitigating climate change and building more environmentally responsible infrastructure.
Section 4: Advanced Concrete Technology: Pushing the Boundaries
The field of concrete technology is continuously evolving, pushing the boundaries of what's possible. Self-consolidating concrete (SCC) and high-performance fiber-reinforced concrete (HPFRC) are examples of cutting-edge advancements. SCC eliminates the need for vibration during placement, making it ideal for complex formwork and congested areas. Its excellent flowability and self-leveling capabilities reduce the labor intensity and enhance the quality of the final product. A case study of a complex dam construction in South America demonstrated the benefits of using SCC in intricate formwork where traditional concrete placement methods were deemed challenging and inefficient. The precise and efficient placement of SCC resulted in significant time and cost savings.
HPFRC combines the high strength of high-performance concrete with the enhanced ductility and fracture toughness of fiber reinforcement. This advanced material is particularly beneficial in structures subjected to dynamic loads or impact events. A case study in earthquake-prone regions in Japan showcased the remarkable performance of HPFRC structures during seismic events. The integration of high-strength fibers significantly improved the energy absorption capacity of the structures, minimizing damage and ensuring their structural integrity during and after the earthquakes.
Furthermore, research into self-healing concrete is progressing steadily. This innovative approach aims to create concrete structures that can automatically repair minor cracks, enhancing their longevity and reducing maintenance costs. This research represents a significant advancement towards developing more resilient and sustainable concrete structures. The use of bio-based materials in concrete production is another active area of research. These materials offer a more sustainable alternative to traditional cementitious materials, reducing the environmental impact of concrete production.
Advanced concrete technology continues to evolve, driven by the need for more durable, sustainable, and high-performance materials. The innovations discussed here represent just a glimpse into the possibilities of this dynamic field. The exploration and implementation of these advanced technologies have the potential to revolutionize the construction industry.
Section 5: The Future of Concrete: Trends and Challenges
The future of concrete is shaped by several key trends, including the increasing demand for sustainable materials, the need for higher performance, and the integration of smart technologies. The adoption of carbon capture and storage technologies in cement production is a crucial step towards reducing the industry's carbon footprint. The development of low-carbon cement alternatives, such as alkali-activated cements, is also gaining momentum. These alternative cements offer the potential for significant reductions in greenhouse gas emissions while maintaining the required performance characteristics.
The integration of digital technologies, such as Building Information Modeling (BIM) and 3D printing, is transforming the design, construction, and management of concrete structures. BIM enables more accurate modeling and simulation, resulting in optimized designs and reduced waste. 3D printing offers the potential for highly customized and complex concrete structures, opening new possibilities in architecture and engineering. A notable example of 3D printing in the construction industry is the creation of innovative architectural structures that feature complex curvatures and intricate designs. The ability to construct these forms would be impossible using conventional construction techniques.
Furthermore, the development of sensors and monitoring systems embedded within concrete structures enables real-time health monitoring and predictive maintenance. This allows for early detection of potential issues, such as cracking or corrosion, preventing major failures and enhancing the longevity of structures. These advancements facilitate the transition towards a data-driven and proactive approach to infrastructure management.
The future of concrete is intertwined with sustainability, technological innovation, and a data-driven approach to design, construction, and management. Addressing the challenges related to carbon emissions and embracing new technologies will be essential for shaping a more sustainable and resilient built environment. The exploration of alternative materials and the integration of smart technologies will play a critical role in shaping the future of concrete and the construction industry as a whole.
Conclusion
Concrete's seemingly simple nature belies its remarkable complexity and potential. By understanding the hidden truths behind aggregate selection, mechanical properties, sustainability, advanced technologies, and future trends, engineers and contractors can unlock its full potential. The continuous evolution of concrete technology promises innovative solutions to address the challenges of building a sustainable and resilient future. Mastering these nuances is paramount for ensuring safer, more durable, and environmentally responsible structures.
The ongoing research and development in this field will undoubtedly lead to even more exciting and sustainable advancements in the years to come. The future of concrete is bright, promising a more sustainable and high-performance future for the built environment. Embracing innovation, prioritizing sustainability, and understanding the hidden complexities of concrete is essential for meeting the challenges of the future.