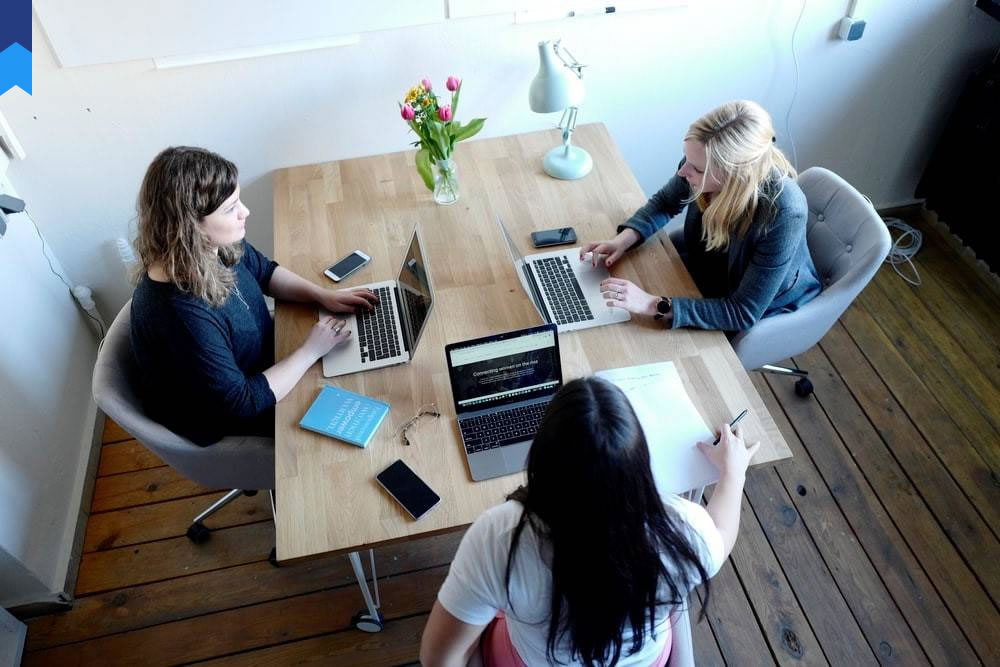
How Best To Master Advanced Welding Techniques With Modern Robotics?
How best to master advanced welding techniques with modern robotics?
Introduction
The welding and fabrication industry is undergoing a significant transformation, driven by advancements in robotics and automation. This shift presents both challenges and opportunities for welders. Mastering advanced welding techniques, coupled with a strong understanding of robotic systems, is becoming increasingly crucial for professionals seeking career advancement and success in this evolving field. This article delves into specific, practical, and innovative aspects of welding and fabrication technology, focusing on how to effectively integrate robotic systems into welding processes to enhance efficiency, precision, and overall quality. We’ll explore various aspects of robotic welding, from programming techniques to safety protocols, and highlight real-world applications showcasing the benefits of this technological integration. The aim is to equip readers with a comprehensive understanding of how to leverage modern robotics to elevate their welding skills and contribute to the future of fabrication.
Programming and Control of Robotic Welding Systems
Effective programming is the cornerstone of successful robotic welding. Understanding the different programming languages and software interfaces used in robotic welding systems is paramount. Many systems employ proprietary software, requiring specific training and expertise. However, the core principles remain consistent: defining the welding path, setting parameters such as welding speed, current, and voltage, and incorporating error detection and correction routines. Advanced programming allows for complex weld geometries and intricate joint configurations, far beyond the capabilities of manual welding. Case study 1: A major automotive manufacturer implemented a robotic welding system for its chassis assembly line, resulting in a 30% increase in production efficiency. This was achieved through optimized programming that minimized non-productive time and maximized weld consistency. Case study 2: A small-scale fabrication shop successfully integrated a collaborative robot (cobot) into its welding operations. The cobot's ease of programming and user-friendly interface enabled the shop to adapt quickly to changing production demands. Furthermore, the cobot’s ability to work alongside human welders improved overall efficiency and reduced workplace injuries.
Beyond basic path programming, advanced techniques such as sensor integration and adaptive control are becoming increasingly prevalent. Sensors allow the robot to compensate for variations in workpiece position and geometry, ensuring consistent weld quality. Adaptive control algorithms dynamically adjust welding parameters based on real-time feedback, further enhancing precision and repeatability. This level of sophistication is particularly crucial in applications requiring high precision and tight tolerances. For example, the aerospace industry relies heavily on robotic welding for critical components due to its ability to produce consistently high-quality welds with minimal defects. Proper programming also encompasses safety considerations, such as implementing emergency stops and collision avoidance systems. These features are vital to ensuring the safety of both the robot and human operators within the welding environment.
Sensor Integration and Quality Control in Robotic Welding
Sensor integration is vital for achieving high weld quality in robotic welding. Various sensors can be incorporated into the robotic system to monitor welding parameters and provide real-time feedback. These include arc sensors, which monitor the welding arc and detect deviations from optimal conditions; seam tracking sensors, which maintain consistent weld position along a joint; and force sensors, which measure the force applied during welding. This data is used to adjust welding parameters dynamically, ensuring consistent weld quality even with variations in material properties or workpiece geometry. Case study 1: A pipeline construction company used sensor-integrated robotic welding to reduce weld defects by 40% and significantly reduce rework. The sensors precisely monitored the weld pool, ensuring consistent penetration and preventing porosity. Case study 2: An electronics manufacturer implemented vision-based seam tracking in its robotic welding system. This technology enabled the robot to precisely follow complex seam geometries, even on irregularly shaped workpieces, leading to improved weld quality and reduced scrap.
The use of advanced sensor technology enables the implementation of sophisticated quality control measures. Real-time data analysis provides valuable insights into weld quality, allowing for proactive adjustments to prevent defects. Furthermore, the data collected can be used for predictive maintenance, allowing for early detection of potential problems and minimizing downtime. This proactive approach significantly reduces the risk of costly weld failures and enhances overall productivity. For example, the data collected by the sensors can be analyzed to identify patterns that may indicate wear and tear on the welding equipment. This information enables maintenance personnel to schedule repairs or replacements before a failure occurs, minimizing production disruption.
Furthermore, integrating advanced imaging systems, such as high-speed cameras, into the robotic welding setup allows for detailed visual inspection of the weld. The images captured can be analyzed to identify potential defects, even those that are not readily detectable through other methods. This non-destructive testing technique greatly enhances the overall quality assurance process. The combination of sensor integration and advanced image analysis provides a comprehensive approach to quality control, ensuring consistently high-quality welds and reducing the risk of failures.
Advanced Welding Processes and Materials
The integration of robotics in welding extends the capabilities of various advanced welding processes, including laser beam welding (LBW), electron beam welding (EBW), and friction stir welding (FSW). LBW is particularly suited for high-precision, deep penetration welds in thin materials. Robotics enhances the precision and repeatability of LBW, making it ideal for applications in aerospace, automotive, and medical device manufacturing. EBW offers even higher precision and deeper penetration capabilities, often used for joining dissimilar metals. The robotic control of the electron beam ensures consistent weld quality and minimizes heat-affected zones. FSW is a solid-state welding process that avoids melting and is suitable for joining difficult-to-weld materials, such as aluminum alloys. Robotic control ensures consistent weld strength and minimizes variations in the weld joint. Case study 1: A manufacturer of high-performance aircraft components used LBW with robotic control to create highly precise and lightweight joints, exceeding the strength requirements specified. Case study 2: A medical device company employed robotic EBW to join stainless steel and titanium, creating highly reliable and biocompatible implants.
Robotics allows for the use of advanced welding materials that are difficult to handle manually. These include high-strength steels, titanium alloys, and composites. The precise control and repeatability offered by robotic systems ensure consistent weld quality, regardless of the material's complexity. This enables manufacturers to use lighter and stronger materials, leading to improvements in product performance and reduced weight. Moreover, robotic welding systems can handle hazardous or difficult-to-weld materials, improving workplace safety. This is particularly important when working with materials that emit toxic fumes or require specialized safety equipment. Furthermore, robotic welding offers higher precision and repeatability when working with complex geometries, which are typical of many advanced materials. This enhances the consistency of the weld and minimizes the risk of defects.
Safety and Maintenance of Robotic Welding Systems
Safety is paramount in any industrial setting, and robotic welding is no exception. Proper safety protocols and training are crucial for minimizing the risk of accidents. This includes implementing appropriate safety measures such as light curtains, emergency stops, and interlocks to prevent unauthorized access to the robot's workspace. Regular maintenance and inspections are necessary to prevent malfunctions that could lead to accidents. Detailed maintenance schedules should be implemented, and qualified personnel should perform regular inspections to identify and address potential problems before they escalate. Case study 1: A company implemented a comprehensive safety program for its robotic welding systems, resulting in a significant reduction in workplace accidents and injuries. The program included regular training for operators, detailed safety protocols, and routine maintenance checks. Case study 2: A manufacturing plant invested in advanced safety features for its robotic welding system, including a sophisticated light curtain system and emergency stops. This enhanced safety measures considerably reduced the risk of accidents and protected the workers from potential hazards.
Regular maintenance includes lubrication, inspection of electrical components, and replacement of worn parts. The use of preventative maintenance reduces downtime and the likelihood of unexpected failures. Advanced diagnostic tools can help identify potential problems early on, allowing for proactive maintenance and reducing the risk of costly repairs. The maintenance process should be documented meticulously, allowing for tracking of maintenance activities and identification of trends. This comprehensive approach to maintenance ensures long-term reliability and safety of the robotic welding system. Furthermore, the development and implementation of robust maintenance procedures are crucial for maximizing the lifespan and effectiveness of the welding robots. This includes not only scheduled maintenance but also prompt addressing of unexpected issues as they arise. A proactive and well-documented maintenance approach minimizes downtime and contributes significantly to the long-term success of implementing robotic welding systems.
Conclusion
Mastering advanced welding techniques with modern robotics requires a multifaceted approach encompassing programming expertise, sensor integration proficiency, understanding of advanced welding processes and materials, and strict adherence to safety protocols. The integration of robotic systems significantly enhances efficiency, precision, and quality in welding and fabrication. By embracing these advancements and continuously updating skills, welders can position themselves for success in this dynamic and evolving industry. The future of welding is intertwined with the capabilities of robotics, promising unprecedented levels of efficiency, precision, and safety. Continuous learning and adaptation are key to thriving in this technologically advanced landscape.