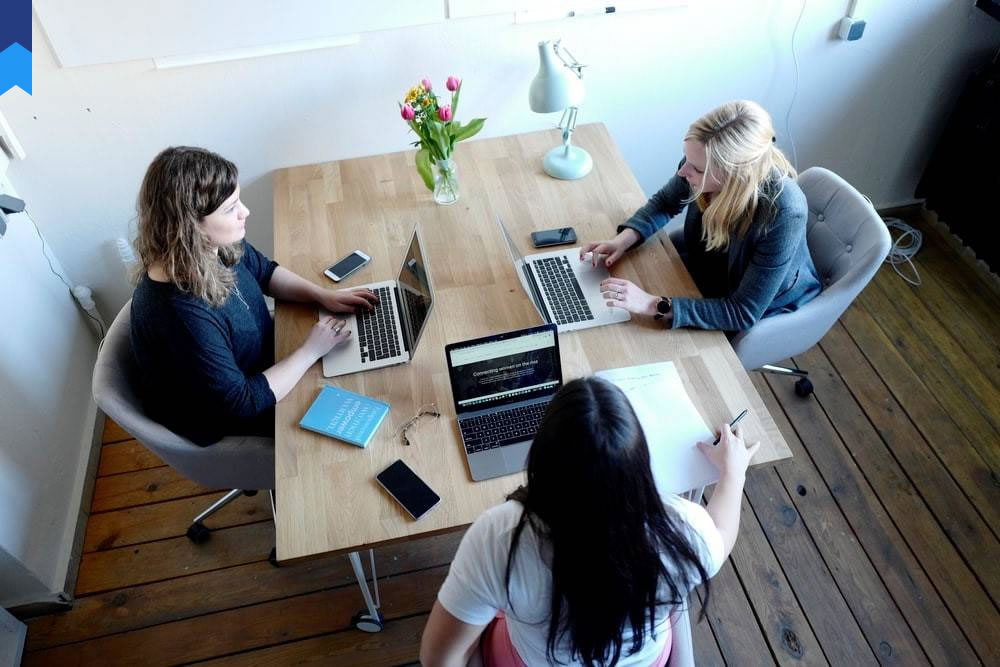
Mastering MIMO Antenna Technology: A Comprehensive Guide
Understanding and effectively utilizing Multiple-Input and Multiple-Output (MIMO) antenna technology is crucial for achieving high-performance wireless communication systems. This comprehensive guide delves into the intricacies of MIMO antennas, exploring their advantages, implementation strategies, and future trends.
MIMO Antenna Fundamentals: A Deep Dive
MIMO technology revolutionizes wireless communication by employing multiple antennas at both the transmitter and receiver ends. This multi-antenna approach allows for simultaneous transmission and reception of multiple data streams, significantly enhancing data rates and system capacity. Unlike single-antenna systems, MIMO leverages spatial multiplexing, which exploits the spatial diversity provided by multiple antennas to create independent communication channels. This means that multiple data streams can be transmitted simultaneously over the same frequency band without interference. Consider a scenario with a single antenna transmitting a signal; if the signal experiences fading, the entire data stream is affected. With MIMO, data is transmitted over multiple paths, reducing the impact of fading and interference on signal quality. The fundamental principle behind MIMO's efficiency lies in its ability to manage signal pathways and mitigate interference, a concept known as spatial diversity. Furthermore, MIMO techniques like beamforming can focus signals toward the receiver, improving signal-to-noise ratio and reducing power consumption.
Case Study 1: The use of MIMO in 4G LTE cellular networks illustrates the technology's impact. By employing multiple antennas, 4G LTE achieves significantly higher data speeds and improved coverage compared to earlier generations of cellular technology. The strategic placement of antennas at both the base station and mobile devices allows for increased spectral efficiency. Case Study 2: The widespread adoption of MIMO in Wi-Fi routers (802.11n, 802.11ac, and 802.11ax) has significantly improved the performance and range of home and office Wi-Fi networks. These standards use MIMO to transmit multiple data streams simultaneously, resulting in faster download and upload speeds, even with multiple devices connected to the network.
Several factors contribute to the successful implementation of MIMO. Antenna spacing is crucial; insufficient spacing can lead to signal correlation and reduced performance gains. Careful antenna design and selection are essential for achieving optimal performance. The use of advanced signal processing techniques is necessary to decode the multiple data streams efficiently. The environment also plays a vital role; the presence of obstacles can affect signal propagation and reduce the effectiveness of MIMO. The overall system design must account for these various factors to fully leverage the benefits of MIMO.
The benefits of MIMO technology are multifaceted. Increased data rates are one of the most significant advantages; MIMO allows for much higher data throughput compared to single-antenna systems, making it ideal for high-bandwidth applications. Improved spectral efficiency is another key advantage; MIMO allows for more efficient use of the available radio spectrum, enabling more users and devices to access the network. Enhanced reliability is also achieved; the use of multiple antennas increases the robustness of the system to fading and interference, leading to more reliable communication. Moreover, the improved coverage provided by MIMO extends the reach of wireless networks and increases their overall availability.
Understanding MIMO Antenna Configurations
Various MIMO antenna configurations exist, each tailored to specific applications and environments. 2x2 MIMO, employing two antennas at both transmitter and receiver, is a common configuration in many wireless devices. 4x4 MIMO, with four antennas on each side, provides even higher data rates and improved reliability. The choice of configuration depends on several factors, including the desired data rates, system complexity, and cost considerations. The spatial diversity afforded by increased antennas contributes to more robust signal transmission. 2x2 MIMO is a good starting point for most applications, providing a significant improvement over single-antenna systems while maintaining reasonable complexity and cost-effectiveness. However, applications requiring very high data rates, such as high-definition video streaming, may benefit from the performance boost of 4x4 MIMO or even higher-order configurations.
Case Study 1: The implementation of 2x2 MIMO in mobile devices has become ubiquitous, providing users with faster data speeds and improved connectivity. This widespread adoption is a testament to the technology's maturity and effectiveness. Case Study 2: The use of 4x4 MIMO in high-performance Wi-Fi routers enables faster data transfer speeds, which is critical for handling multiple devices and high-bandwidth applications like 4K video streaming. This reflects the adaptability of MIMO technology to various demands.
Antenna placement is crucial for optimal performance. Antennas should be spaced sufficiently apart to minimize signal correlation; otherwise, the benefits of spatial diversity are diminished. The specific spacing requirements depend on the frequency of operation and the environment. Proper antenna orientation also affects signal quality. In some cases, smart antenna systems adapt their orientation dynamically based on signal conditions, optimizing performance. This requires sophisticated signal processing and control systems.
Signal processing techniques play a critical role in MIMO systems. Techniques like beamforming, space-time coding, and maximum-likelihood detection are used to enhance data rates, reliability, and spectral efficiency. Beamforming focuses the transmitted signal towards the receiver, reducing signal scattering and interference. Space-time coding combines data streams across antennas, enhancing reliability in fading environments. Maximum-likelihood detection is employed to decode the multiple data streams received at the receiver, ensuring reliable data recovery.
Advanced MIMO Techniques: Beamforming and Spatial Multiplexing
Beamforming is a key technique in MIMO systems that steers the transmitted signal toward the intended receiver. By adjusting the phase and amplitude of signals across multiple antennas, beamforming concentrates energy in a specific direction, improving signal strength and reducing interference. Adaptive beamforming adjusts the beam direction dynamically based on channel conditions, optimizing performance in varying environments. This adaptability is critical for wireless systems operating in dynamic environments where obstacles and interference sources may change constantly. The use of digital beamforming, enabled by advancements in digital signal processing, provides a flexible approach for optimizing signal transmission. The resulting improvement in signal quality and coverage significantly improves overall system performance.
Case Study 1: Beamforming is extensively used in 5G cellular networks to provide highly focused signal transmission, maximizing data rates and improving coverage. This is particularly critical in dense urban environments with significant signal interference. Case Study 2: Wi-Fi 6 (802.11ax) employs beamforming to improve signal strength and reach, enhancing Wi-Fi performance in homes and offices with many connected devices.
Spatial multiplexing is another crucial aspect of MIMO, allowing for the simultaneous transmission of multiple data streams over the same frequency band. This is achieved by creating independent spatial channels through the use of multiple antennas. Each data stream is transmitted through a different spatial channel, enabling high data throughput. However, the effectiveness of spatial multiplexing depends on the characteristics of the wireless channel, such as multipath propagation and fading. Advanced signal processing techniques are required to efficiently decode the multiple streams and mitigate interference. The proper design and placement of antennas play a critical role in maintaining the independence of the spatial channels. Incorrect antenna placement can lead to channel correlation and reduce the effectiveness of spatial multiplexing.
Orthogonal frequency-division multiplexing (OFDM) is frequently combined with MIMO to further enhance performance. OFDM divides the wideband signal into multiple narrowband subcarriers, allowing for robust data transmission in multipath environments. Combining OFDM with MIMO leverages the benefits of both techniques, achieving significantly higher data rates and improved spectral efficiency. This combination provides robustness against multipath fading and intersymbol interference, crucial factors in modern wireless communication systems. The joint design of OFDM and MIMO considers the interaction between these two techniques, optimizing overall system performance.
MIMO Antenna Design and Implementation Considerations
Designing and implementing MIMO antenna systems requires careful consideration of various factors, ensuring optimal performance. Antenna element selection is paramount. Different antenna types, such as patch antennas, dipole antennas, and microstrip antennas, each have unique characteristics affecting performance in different environments. The choice of antenna type is influenced by factors such as frequency, size, cost, and radiation pattern. Careful consideration of these factors is essential for effective system design.
Case Study 1: The use of patch antennas in many mobile devices allows for compact design and efficient integration within the device's form factor. Case Study 2: The use of specialized antenna arrays in high-performance base stations provides highly directional transmission, optimizing coverage and capacity.
Antenna spacing is critical for achieving spatial diversity. Insufficient spacing leads to strong signal correlation, diminishing the benefits of MIMO. The optimal spacing depends on the operating frequency and environment. Simulations and measurements are often employed to determine the optimal spacing in a given scenario. This process involves analyzing the correlation between signals from different antennas to find the spacing that minimizes correlation while maintaining acceptable antenna size and form factor.
Signal processing techniques play a critical role in MIMO system performance. Techniques such as channel estimation, equalization, and decoding are essential for accurately recovering the transmitted data streams. Channel estimation determines the characteristics of the wireless channel, while equalization compensates for channel impairments. Decoding algorithms are employed to recover the original data from the received signals. Advanced signal processing techniques are often required for optimal performance in challenging wireless environments.
Future Trends in MIMO Antenna Technology
The future of MIMO antenna technology is marked by ongoing advancements in various areas. Massive MIMO, employing a large number of antennas, is set to significantly enhance data rates and capacity. This technology enables more efficient use of the available spectrum, supporting a vast number of users and devices. However, the increased complexity and cost of massive MIMO systems require advanced signal processing and hardware solutions. The use of advanced signal processing techniques and hardware is expected to address these challenges. Furthermore, the development of integrated antenna modules is streamlining the implementation of MIMO in various applications.
Case Study 1: The deployment of massive MIMO in 5G and beyond networks will play a significant role in meeting the growing demand for high-speed wireless data. Case Study 2: Integration of MIMO antennas within mobile devices is leading to more compact and efficient designs.
The integration of artificial intelligence (AI) and machine learning (ML) in MIMO antenna systems is expected to further optimize performance. AI and ML algorithms can dynamically adapt antenna parameters and signal processing techniques based on real-time channel conditions, maximizing data rates and reliability. This intelligent adaptation is crucial in dynamic environments where channel conditions are constantly changing. The use of AI and ML offers significant potential for optimizing various aspects of MIMO systems, including beamforming, channel estimation, and resource allocation.
The development of reconfigurable antennas allows for dynamic adjustments to the antenna parameters, further improving performance in varying environments. Reconfigurable antennas adapt to changing channel conditions, maximizing performance. This adaptability is crucial for mobile devices operating in dynamic environments with varying signal conditions. Future research focuses on developing more efficient and cost-effective reconfigurable antenna systems that adapt rapidly to changing environments.
Conclusion
MIMO antenna technology has revolutionized wireless communication, offering significant improvements in data rates, spectral efficiency, and reliability. Understanding the fundamentals, configurations, advanced techniques, and implementation considerations is crucial for maximizing the benefits of this technology. The future trends in MIMO, including massive MIMO, AI/ML integration, and reconfigurable antennas, promise further enhancements in wireless communication capabilities. As wireless networks continue to grow in complexity and demand, MIMO will play an increasingly vital role in enabling high-performance wireless systems.
The ongoing advancements in MIMO technology highlight the ongoing quest to improve wireless communications. The continued research and development in this field will continue to reshape the landscape of wireless connectivity, driving improvements in speed, reliability, and capacity. The potential of MIMO is far from fully realized, with future developments promising even greater efficiency and capabilities in wireless systems.