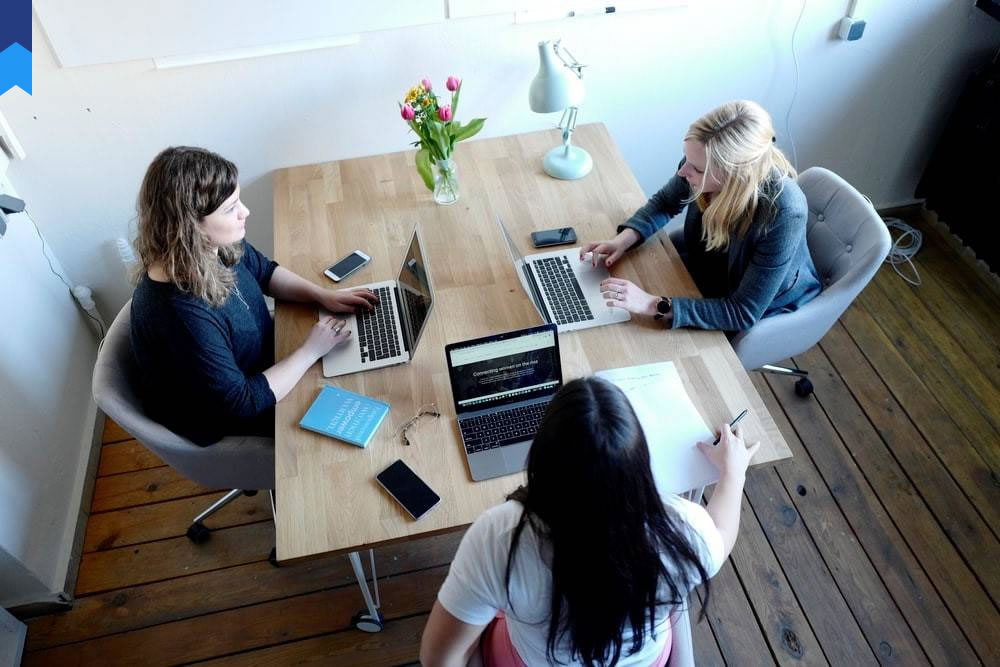
Smart Pulse Circuit Decisions
Pulse circuits, the digital heartbeats of countless electronic devices, often present design challenges that demand innovative solutions. This article delves into specific, practical, and innovative aspects of pulse circuit design, moving beyond basic overviews and challenging conventional wisdom.
Understanding Pulse Circuit Fundamentals
Before diving into advanced techniques, it's crucial to grasp fundamental concepts. Pulse circuits rely on short, discrete pulses of electrical energy to transmit information or control operations. These pulses can be characterized by their amplitude, duration, rise and fall times, and repetition rate. Understanding these parameters is critical for effective design.
One key aspect is choosing the appropriate pulse generation method. Options include using astable multivibrators, monostable multivibrators, or specialized integrated circuits. Each method offers different trade-offs regarding precision, stability, and complexity. For instance, a 555 timer IC provides a simple and versatile solution for generating pulses with adjustable duration, while a more complex circuit using operational amplifiers might offer better precision for demanding applications.
Case Study 1: A medical device manufacturer needed a highly precise pulse generator for a pacemaker. Using a specialized IC with low jitter and high stability, they ensured reliable and consistent heart stimulation, significantly improving device performance and patient safety. Case Study 2: In industrial automation, a simple 555 timer-based circuit was used to generate control pulses for a robotic arm. Its simplicity and low cost made it an ideal choice for this application.
Another crucial consideration is pulse shaping. Often, the raw pulses generated require shaping to meet specific requirements. This can involve filtering, amplification, or using specialized shaping circuits to control the pulse rise and fall times. Proper pulse shaping is essential for preventing signal distortion and ensuring reliable operation, especially in high-speed communication systems.
The selection of appropriate components, including resistors, capacitors, and transistors, directly influences pulse characteristics. Careful component selection, considering tolerance and temperature stability, is vital for achieving desired pulse parameters and ensuring long-term reliability. This includes using precision components in high-accuracy applications or employing temperature compensation techniques where necessary.
Furthermore, proper grounding and shielding techniques are indispensable. These reduce unwanted noise interference and prevent signal degradation. Careful layout and grounding practices help ensure the integrity of the pulse signal throughout the circuit.
Finally, simulation software plays a vital role in optimizing pulse circuit design. Using simulation tools allows designers to model circuit behavior, test different configurations, and identify potential issues before physical prototyping. This reduces development time and minimizes errors in the final design.
Advanced Pulse Shaping Techniques
Beyond basic pulse generation, advanced shaping techniques can significantly enhance circuit performance. Techniques like pulse width modulation (PWM) allow precise control over the average power delivered to a load by varying the pulse width while maintaining a constant frequency. PWM is extensively used in motor control systems, LED dimming, and power converters.
Another powerful technique is pulse compression, which increases the peak power of a pulse by shortening its duration. This finds application in radar systems and high-energy pulsed lasers. Conversely, pulse stretching increases pulse duration, which is beneficial in certain types of signal processing and communication systems where longer pulse durations are advantageous.
Case Study 3: An automotive manufacturer utilized PWM to efficiently control the speed of electric motors in hybrid vehicles. This allowed for precise control, improved energy efficiency and extended battery life. Case Study 4: Researchers are exploring pulse compression techniques to enhance the resolution of medical imaging systems, leading to clearer and more detailed images.
The choice of pulse shaping technique often depends on the specific application. High-speed digital systems may require sharp, well-defined pulses with minimal jitter, while low-power applications might prioritize energy efficiency. Careful consideration of these factors is crucial in selecting the most appropriate technique.
Moreover, modern digital signal processing (DSP) techniques offer sophisticated pulse shaping capabilities. DSP algorithms can be used to filter noise, compensate for distortion, and create custom pulse shapes to meet very specific application requirements. This allows for greater flexibility and control in pulse circuit design.
Furthermore, the integration of microcontrollers and programmable logic devices (PLDs) allows for highly flexible and adaptable pulse generation. These programmable components offer significant design flexibility, enabling the creation of custom pulse shapes and sequences in response to various inputs. This is highly beneficial in applications where adaptability is critical.
The use of advanced materials and components can also significantly improve pulse circuit performance. High-speed transistors and advanced packaging technologies enable faster switching speeds and better signal integrity. This is particularly important in high-frequency and high-speed applications.
Designing for Noise Immunity
Pulse circuits are susceptible to noise interference, which can lead to malfunctions or data errors. Designing for noise immunity is crucial for reliable operation. Techniques include proper grounding, shielding, and the use of noise filters. These help minimize unwanted noise signals from affecting the pulse signals.
Careful component selection is also important. Using components with low noise characteristics, such as low-noise operational amplifiers and precision resistors, can significantly reduce noise levels. Furthermore, using differential signaling, a technique where two signals carrying the same information but with opposite polarities, helps reject common-mode noise.
Case Study 5: A telecommunications company designed a pulse-based communication system for a noisy environment, implementing robust shielding and filtering to maintain signal integrity. Case Study 6: In industrial control systems, a proper grounding scheme was implemented to avoid ground loops and minimize interference, guaranteeing dependable system operation despite a harsh electrical environment.
Another effective technique is using opto-isolators. These devices transfer signals using light, providing electrical isolation and preventing noise from propagating between different parts of the circuit. This isolation is crucial in applications where high voltage or strong electromagnetic fields are present.
Moreover, employing error detection and correction codes can enhance system resilience against noise. By adding redundant information to the pulse signals, these codes enable the detection and correction of errors caused by noise, ensuring data integrity. This technique is commonly used in data storage and communication systems.
Furthermore, designing for sufficient signal-to-noise ratio (SNR) is critical. The SNR represents the ratio of the desired signal power to the noise power. A high SNR indicates a strong signal relative to the noise, improving the reliability of the system. Maintaining an adequate SNR is crucial for accurate and reliable signal transmission.
Finally, thorough testing and validation are essential to ensure that the pulse circuit is sufficiently immune to noise. This involves subjecting the circuit to various noise conditions during testing to verify its performance and robustness in real-world scenarios. Thorough testing is paramount for achieving reliable operation.
Optimizing Power Consumption
In many applications, minimizing power consumption is a critical design goal. Techniques for optimizing power consumption in pulse circuits include using low-power components, employing efficient switching techniques, and implementing power management strategies.
Choosing low-power integrated circuits and transistors significantly reduces power consumption. These components are designed to operate efficiently at low voltages and currents, resulting in reduced energy consumption. Utilizing energy-efficient components is pivotal for low-power applications.
Case Study 7: A portable medical device manufacturer reduced power consumption by using low-power microcontrollers and optimized pulse generation techniques. Case Study 8: In the design of wireless sensor networks, low-power components and energy harvesting techniques are crucial for extending battery life.
Efficient switching techniques, such as using MOSFETs instead of bipolar transistors in some applications, can dramatically reduce power loss. MOSFETs generally have lower on-resistance and faster switching times, leading to reduced power dissipation during switching events. Careful selection of switching elements is vital for energy efficiency.
Furthermore, implementing power management strategies, such as using sleep modes or duty cycling, allows the circuit to operate at reduced power levels when not actively processing pulses. This involves strategically managing the active periods and periods of inactivity, resulting in power savings.
Moreover, optimizing the pulse width and frequency can influence power consumption. Shorter pulses or lower frequencies generally result in reduced power dissipation, but a careful balance must be struck to ensure the functionality and performance requirements of the system are met. This balance between power efficiency and performance needs thorough evaluation.
Additionally, innovative power management integrated circuits (PMICs) offer sophisticated power control capabilities. These PMICs integrate multiple power management functions, such as voltage regulators, battery chargers, and power switches, optimizing energy efficiency and simplifying the design.
Finally, advanced packaging techniques can also reduce power consumption by minimizing parasitic capacitances and resistances within the circuit. This leads to lower power dissipation and improves the overall efficiency of the pulse circuit. Optimal packaging is also essential for power management.
Emerging Trends and Future Directions
The field of pulse circuit design is constantly evolving, with new trends and technologies emerging continuously. One significant trend is the increasing use of high-speed, low-jitter pulse generation techniques for high-bandwidth communication systems. This requires the use of advanced components and sophisticated circuit design techniques.
Another important trend is the integration of pulse circuits with other technologies, such as microelectronics and photonics, to create more complex and powerful systems. This integration leads to devices with enhanced functionality and improved performance capabilities.
Case Study 9: Research is being conducted on the use of optical pulses in high-speed data communication, allowing for much higher data rates than traditional electronic pulse-based systems. Case Study 10: Researchers are investigating the application of pulse circuits in neuromorphic computing, emulating biological neural networks for energy-efficient artificial intelligence applications.
The development of new materials and fabrication techniques is also driving advancements in pulse circuit design. For instance, the use of advanced semiconductor materials allows for the creation of faster and more energy-efficient devices. Material science innovation significantly contributes to the advancement of pulse circuit technology.
Furthermore, machine learning techniques are being applied to pulse circuit design to optimize performance and reduce development time. Machine learning algorithms can analyze large datasets of circuit simulations to identify optimal design parameters and predict circuit behavior.
Moreover, the exploration of novel pulse shaping techniques, such as those using artificial neural networks (ANNs), has the potential to enhance the precision and flexibility of pulse generation. ANN-based pulse shaping algorithms can adapt to changing conditions and optimize pulse parameters in real-time.
Finally, the push towards miniaturization and integration is driving the development of smaller, more power-efficient pulse circuits. This miniaturization is crucial for applications in wearable electronics and implantable medical devices, where size and power consumption are critical constraints.
Conclusion
Designing effective pulse circuits requires a multifaceted approach, encompassing fundamental understanding, advanced techniques, and a keen awareness of emerging trends. By carefully considering factors such as pulse shaping, noise immunity, and power consumption, designers can create reliable, efficient, and high-performance pulse circuits for a wide range of applications. The continuous evolution of this field promises even more innovative solutions in the future, driving advancements in various technological sectors.
From the fundamental principles of pulse generation to the intricacies of advanced shaping techniques and noise reduction strategies, this exploration has highlighted the critical design considerations for achieving optimal pulse circuit performance. Understanding and addressing these aspects are paramount for creating robust and efficient systems in diverse applications. The future of pulse circuits holds exciting possibilities with ongoing research and development in areas such as high-speed communication and neuromorphic computing shaping the next generation of electronic devices and systems.