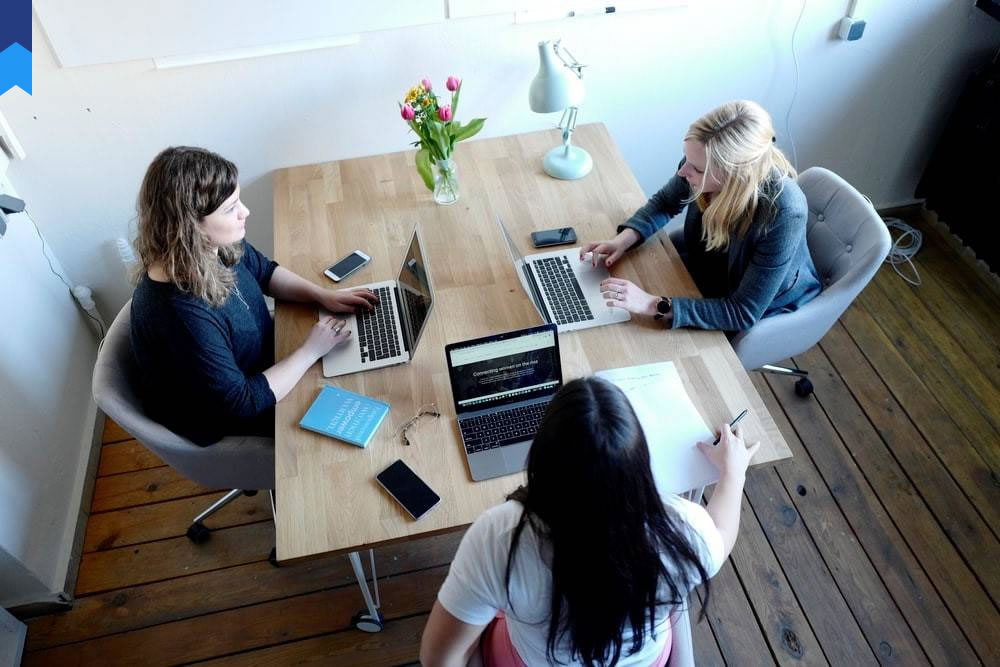
Stop Chasing Signal Strength: The Unexpected Downsides Of Antenna Optimization Obsession
Antenna design is a crucial element in any wireless communication system. However, a singular focus on maximizing signal strength can lead to unforeseen consequences, hindering overall system performance and efficiency. This article explores the pitfalls of obsessive antenna optimization, presenting alternative approaches that prioritize holistic system design over singular metrics.
Antenna Optimization's Shadow Side: Beyond Signal Strength
The relentless pursuit of maximum signal strength often overshadows other crucial antenna characteristics. While a strong signal is undeniably important, ignoring factors like antenna gain, beamwidth, polarization, and impedance matching can severely compromise performance. A narrow beamwidth, for example, might provide exceptional signal strength in a specific direction but leave significant areas with poor coverage. Similarly, a mismatch in impedance between the antenna and the transmission line can result in signal reflection and loss, negating the benefits of high signal strength. Consider the case of a cellular tower optimized solely for peak signal strength. While the strongest signals are in one area, other areas might experience coverage gaps despite overall high signal power from the antenna.
Moreover, focusing solely on signal strength can drive up costs unnecessarily. Employing high-power amplifiers and sophisticated antenna arrays might boost signal strength, but this adds to capital expenditure and ongoing operational costs. A more nuanced approach considers the trade-offs between signal strength and cost-effectiveness. For instance, a properly designed network of smaller, lower-power antennas could potentially provide better coverage than a single high-powered antenna, at a lower cost. Case study: A rural telecommunication company found that deploying a distributed network of lower-gain antennas improved overall coverage and reliability while reducing capital costs by 15% compared to a high-power antenna based approach.
Furthermore, environmental factors frequently influence signal strength. Obstacles like buildings, trees, and even weather conditions can significantly affect signal propagation, rendering high signal strength readings unreliable. This necessitates a holistic approach where the impact of the surrounding environment on signal transmission is considered alongside antenna characteristics. An example is a university campus with many dense buildings. An antenna optimized for maximum signal strength within a single building might not deliver the same result across the entire campus. A more effective approach could include deploying multiple low-power antennas strategically placed to mitigate signal interference from the buildings.
Finally, the regulatory landscape plays a significant role. Strict regulations on electromagnetic emissions limit the maximum power that can be used, thus constraining the signal strength achievable by an antenna. Ignoring these regulations can lead to legal repercussions and system shutdowns. For instance, a company attempting to maximize signal strength might inadvertently exceed emission limits, causing severe fines or operational suspension. It is therefore essential to incorporate regulatory compliance into antenna design.
The Holistic Approach: A Systems Perspective
A comprehensive approach to antenna design requires a shift in perspective from a singular focus on signal strength to a holistic consideration of the entire system. This encompasses understanding the specific needs of the application, optimizing for relevant metrics beyond just signal strength and designing for robustness and resilience. An example would be a smart home system using multiple devices. Each device may require a specific antenna design tailored to the type of signal and required range. Maximizing signal strength in one part of the system might disrupt another.
This requires taking into account the entire communication channel, including the transmitter, the receiver, and the propagation medium. Careful consideration should be given to factors like path loss, multipath interference, and fading effects, in addition to the antenna's characteristics. Designing for resilience involves accounting for unexpected conditions, such as equipment failure or adverse weather. For instance, in a rural area, a communication system should be designed to function during heavy rainfall, while in urban environments it should handle signal interference from adjacent wireless systems. The case study of a major mobile network operator illustrates this: They designed redundant antennas at each cell tower to automatically switch to a backup antenna during temporary equipment failures.
Moreover, a systems perspective involves analyzing the interplay between various antenna characteristics. For example, optimizing for high gain might necessitate a narrower beamwidth, which could then negatively impact coverage. A balanced approach is necessary to achieve optimal performance for the given application. For instance, in a crowded urban area, a wide beamwidth, though possibly delivering a weaker signal, could deliver far better coverage than a higher-gain, narrow-beam antenna.
Furthermore, this approach necessitates a thorough understanding of the intended application's specific requirements. Different applications, such as long-range communication, high-data-rate transmission, or high-mobility environments, have different antenna requirements. A one-size-fits-all approach to antenna optimization is ill-advised. One application, like a long-range point-to-point link, would benefit more from directional antennas, while a wireless network in an office building would benefit from antennas with wide coverage and lower power to reduce interference.
Beyond Signal Strength: Exploring Alternative Metrics
Moving beyond the simplistic pursuit of maximum signal strength requires identifying and optimizing for alternative, often more relevant metrics. These include effective isotropic radiated power (EIRP), signal-to-noise ratio (SNR), bit error rate (BER), and coverage area. EIRP considers both the antenna gain and the transmitter power, providing a more holistic measure of signal strength. SNR assesses the quality of the received signal by comparing the signal power to the noise power; a higher SNR generally translates to better signal quality. BER quantifies the number of errors in data transmission, and a low BER is crucial for reliable communication. Coverage area, which measures the geographic region adequately served by an antenna, directly addresses the practicality of an antenna’s performance.
Optimizing for these metrics often involves complex simulations and modeling. Software tools like FEKO, CST Microwave Studio, and HFSS allow engineers to simulate antenna performance under various conditions, predict coverage patterns, and identify areas for improvement. These simulations are crucial for identifying optimal antenna placement, design, and parameters without the need for extensive physical prototyping. Consider the case of an aerospace company deploying antennas on a satellite. Extensive simulation was used to ensure the antennas could withstand the harsh space environment and provide reliable communication across vast distances.
Furthermore, the choice of antenna type significantly impacts these metrics. Different types of antennas, such as dipole antennas, patch antennas, and horn antennas, offer different characteristics in terms of gain, beamwidth, and polarization. Selecting the appropriate antenna type is essential for achieving optimal performance. The selection would depend on factors like frequency, environment, and required coverage. For instance, a long-range communication system might use a parabolic dish antenna for high gain and directivity, while a short-range application might use a simple dipole antenna.
Finally, the integration of advanced signal processing techniques can help compensate for limitations in antenna performance. Techniques like adaptive beamforming and equalization can improve signal quality and increase system capacity. For instance, an adaptive beamforming system could automatically adjust the antenna’s beam pattern to focus on the strongest signal, while equalization could compensate for signal distortions caused by multipath interference.
Innovative Antenna Technologies: Redefining the Landscape
The field of antenna technology is constantly evolving, with new innovations constantly pushing the boundaries of what’s possible. These innovations address many of the limitations of traditional antenna designs and offer new opportunities to optimize performance in various applications. Reconfigurable antennas, for example, can dynamically adjust their characteristics in response to changing environmental conditions or operating requirements. This allows for optimized performance in diverse scenarios without requiring multiple antennas. For example, a reconfigurable antenna for a mobile device could adjust its beam pattern to focus on the strongest cell tower signal, improving reception and reducing power consumption.
Metamaterials, with their ability to manipulate electromagnetic waves in novel ways, are opening up new possibilities in antenna design. These materials can be used to create compact antennas with enhanced performance or to create antennas with unusual radiation patterns. Imagine a metamaterial antenna embedded within a wall that provides efficient signal transmission without affecting the aesthetic of the building. Case study: researchers have developed metamaterial antennas for enhancing 5G cellular networks, providing improved coverage and capacity in dense urban areas. The use of such metamaterials in antenna design offers significantly improved performance metrics.
Another significant advancement is the integration of antenna technology with other technologies. For example, integrating antennas into printed circuit boards (PCBs) enables the creation of compact and cost-effective antenna solutions for various applications. This approach is particularly relevant for miniature wireless devices where space is at a premium. Integrating antennas directly into device components eliminates the need for external antennas, simplifies the design, and reduces the overall size and weight. For example, many modern smartphones use antennas integrated directly into their casing, improving design flexibility.
Finally, advancements in antenna array technology have led to the development of more efficient and powerful antenna systems. Large antenna arrays can be used to create highly directional beams with exceptional gain and low side lobes, enabling high-data-rate communication over long distances. This is particularly important for applications such as satellite communication and radar systems. For example, the next generation of 5G cellular base stations utilizes massive MIMO (multiple-input and multiple-output) antenna arrays for improved capacity and coverage.
Conclusion
Antenna optimization is essential, but a singular focus on signal strength can be detrimental to overall system performance. By adopting a holistic approach that considers diverse metrics, environmental factors, and regulatory compliance, designers can create more robust and efficient communication systems. Exploring innovative technologies such as reconfigurable antennas, metamaterials, and antenna arrays offers further opportunities to optimize performance and expand the capabilities of wireless communication. The future of antenna design lies in understanding the complexities of the system and striking a balance between various performance metrics, rather than solely pursuing maximum signal strength. A shift towards this holistic approach is crucial for future wireless innovations.