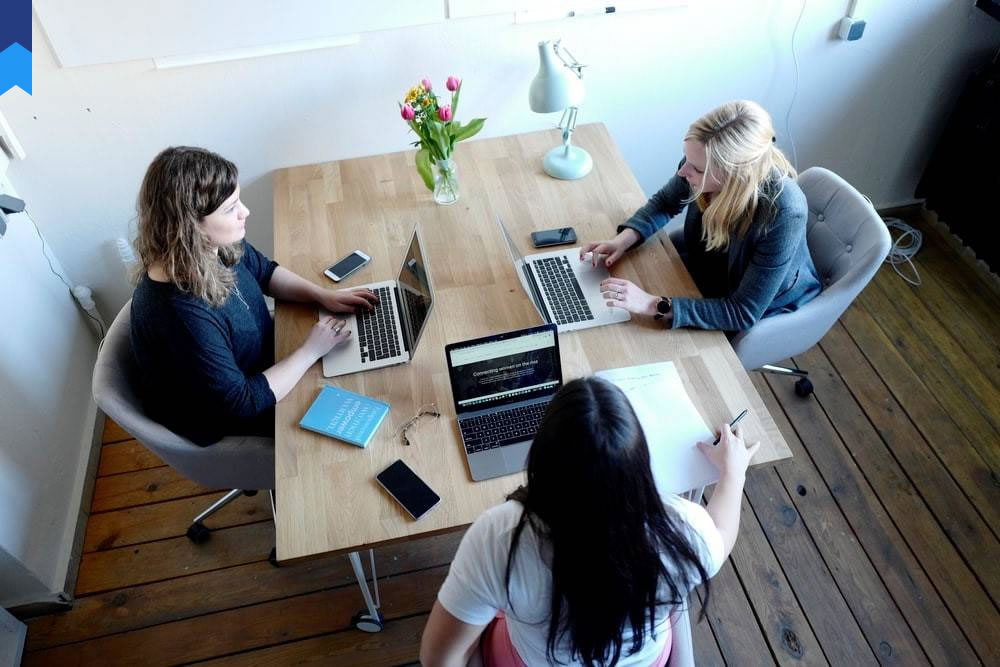
The Reality Behind Microfabrication's Hidden Challenges
Microfabrication, the art of crafting incredibly tiny structures, holds immense promise for technological advancement. From revolutionizing medical diagnostics to enhancing computing power, its impact is undeniable. However, the journey from concept to functional device is far from straightforward. This article delves into the often-overlooked realities of microfabrication, exposing the complexities and challenges that lie beneath the surface of this seemingly miraculous technology.
Mastering the Microscale: Precision and Control
Microfabrication demands an unprecedented level of precision. Features measured in micrometers or even nanometers require incredibly sophisticated tools and techniques. Cleanroom environments are essential to prevent contamination, and even the slightest vibration or temperature fluctuation can impact the final product. Consider the fabrication of microfluidic devices, where channels only a few micrometers wide are etched into silicon. Any deviation from the design can render the device useless. One example is the development of lab-on-a-chip devices, where precise control over fluid flow is critical for diagnostic applications. A slight imperfection in the channel geometry can lead to inaccurate measurements. Similarly, the production of microelectromechanical systems (MEMS) requires incredibly precise alignment and etching processes to create functional components at the microscale. A case study of MEMS gyroscopes shows the criticality of control, as any misalignment can result in significant errors in angular rate measurement.
Maintaining consistent quality across large-scale production is another major hurdle. Even with advanced automation, variations in material properties, process parameters, and environmental conditions can lead to inconsistencies. Statistical process control methods are employed to monitor and minimize these variations, but achieving truly uniform outcomes remains a challenge. For instance, in the production of microarrays for genomic research, the consistent spot size and density are crucial for accurate and reliable data. Any deviations can affect the results of gene expression analysis. Another example is in the manufacturing of semiconductor chips, where even minute variations in transistor dimensions can impact performance and yield. Stringent quality control and sophisticated metrology techniques are crucial to maintain high yields in these high-volume production settings.
Furthermore, the development of new materials and processes for microfabrication is an ongoing endeavor. Researchers continually strive to improve resolution, reduce costs, and expand the range of materials that can be used. For instance, the development of novel polymers for microfluidic devices with improved biocompatibility is a major area of research. Exploring new etching techniques for advanced semiconductor manufacturing is another significant area of focus. The quest for smaller, faster, and more energy-efficient electronics drives continuous innovation in materials science and process engineering. A case study of the development of novel photoresists for nanoimprint lithography illustrates the importance of material innovation in advancing microfabrication capabilities. This highlights the intricate balance between material selection, process control, and the final device performance.
The integration of multiple functionalities on a single microscale device presents another significant challenge. This requires precise control over the layering and patterning of different materials, often involving complex and multi-step processes. For example, the development of lab-on-a-chip devices often involves the integration of microfluidic channels, sensors, and actuators on a single substrate. These processes demand highly advanced fabrication techniques and precise alignment procedures to ensure proper functionality. Another example is the development of three-dimensional microstructures, which requires specialized techniques such as two-photon polymerization or layer-by-layer deposition. These techniques offer increased design flexibility but also add to the complexity of the fabrication process.
The Cost Conundrum: Balancing Innovation and Affordability
Microfabrication can be incredibly expensive. The specialized equipment, cleanroom facilities, and highly trained personnel required represent a significant investment. This cost can be a major barrier to entry for smaller companies and research groups. Cost-effective fabrication techniques are a constant area of research. For example, advancements in additive manufacturing techniques offer the potential for cheaper and faster prototyping. However, the resolution and precision of these methods may still lag behind traditional techniques for certain applications. A case study of a start-up attempting to commercialize a novel microfluidic device highlights the challenges of managing costs while maintaining quality. This case study also shows how creative solutions can be employed, such as outsourcing certain manufacturing steps, to decrease the overall cost of production.
The high cost also limits the accessibility of microfabrication technology to researchers and developers in resource-constrained settings. Developing cheaper alternatives without compromising on performance remains a major challenge. One example is the exploration of low-cost materials and processes for use in developing countries. Research efforts are focused on making these technologies more accessible to global communities. Another important case is the exploration of open-source microfabrication tools and techniques, aiming to make the technology more accessible to individuals and smaller teams. The goal is to democratize access to this advanced technology, empowering more people to innovate in this field.
Furthermore, optimizing the fabrication process for mass production is key to reducing costs. This involves careful process design, automation, and efficient utilization of resources. However, this process optimization can be complex and time-consuming, requiring detailed analysis and simulation. A case study of a company that successfully scaled up the production of a specific microdevice illustrates the importance of process optimization. This company's experience highlights the iterative nature of process optimization and the need for continuous improvement. The implementation of lean manufacturing principles and automation can help significantly reduce production costs while maintaining quality.
The challenge extends beyond the immediate costs of fabrication. There are also significant costs associated with design, prototyping, testing, and characterization. These costs can be particularly high for complex devices. This requires careful planning and resource allocation. A case study of a research project using advanced microfabrication techniques highlights the importance of thorough planning and effective management of resources to minimize costs. The strategic use of simulations and modeling can help reduce prototyping iterations and save valuable time and resources, decreasing the overall cost of the project.
Bridging the Gap: From Lab to Market
Translating laboratory prototypes into commercially viable products is a significant hurdle. Scaling up production, ensuring reliability, and meeting regulatory requirements all present challenges. The transition from small-scale research to large-scale manufacturing requires significant expertise and resources. One example is the difficulties faced by medical device companies in obtaining regulatory approval for microfluidic diagnostic devices. Strict regulations require rigorous testing and validation, which can be a lengthy and costly process. Another example is the challenges encountered by companies developing microelectronic components that must meet stringent performance and reliability standards.
Reliability and durability are critical for many microfabricated devices, especially in demanding environments. The long-term performance and stability of these devices must be thoroughly characterized and validated. This requires extensive testing and analysis. A case study of the failure analysis of a specific microdevice shows the importance of rigorous reliability testing and the need for robust design methodologies. This case study underscores the need for proactive reliability management throughout the design and manufacturing process. The use of advanced simulation techniques and accelerated life testing can help to identify potential weaknesses and improve the reliability of the devices.
Meeting industry standards and regulations is essential for commercial success. Different industries have specific requirements regarding safety, performance, and biocompatibility. This can add complexity to the design and manufacturing process. A case study of a company that successfully navigated the regulatory approval process for a new medical device highlights the importance of understanding and complying with all relevant regulations. This case study underscores the importance of early engagement with regulatory bodies and the necessity of well-documented testing procedures. This requires careful planning and potentially significant investments in testing and documentation.
Finally, the integration of microfabricated devices into larger systems presents another challenge. The device must interface seamlessly with other components and meet the overall system requirements. This can be a complex engineering challenge, requiring expertise in diverse fields. A case study of a company that successfully integrated a microfluidic sensor into a larger analytical system highlights the importance of systems-level thinking and the need for close collaboration between different engineering disciplines. This case study underscores the need for a holistic approach that considers the device's performance in the context of the entire system. This requires a deep understanding of both the device itself and its intended application within the larger system.
Emerging Trends and Future Directions
Microfabrication is a rapidly evolving field. Advances in nanotechnology, materials science, and process engineering are continually pushing the boundaries of what is possible. The development of new lithographic techniques, such as extreme ultraviolet (EUV) lithography, allows for the fabrication of even smaller features. This opens up possibilities for more powerful and efficient microelectronic devices. Furthermore, advancements in additive manufacturing techniques are expanding the range of materials and geometries that can be fabricated. A case study of a laboratory demonstrating the fabrication of complex three-dimensional microstructures using two-photon polymerization highlights the potential of this emerging technology. This technology has broad implications for applications in tissue engineering, microfluidics, and other fields.
The increasing use of artificial intelligence (AI) and machine learning (ML) in microfabrication is transforming the design and optimization of processes. AI-powered tools can analyze large datasets, predict process outcomes, and automate process control. This can lead to significant improvements in efficiency, yield, and quality. A case study of a semiconductor manufacturer using AI for process control highlights the potential benefits of this technology. This case study shows how AI-powered algorithms can significantly reduce the number of defects and improve overall yield. The use of AI and ML is expected to play an increasingly important role in the development of future microfabrication technologies.
The integration of microfabrication with other technologies, such as microelectronics and photonics, is creating opportunities for new and innovative devices. For example, the integration of microfluidic devices with optical sensors allows for highly sensitive and compact analytical systems. Another emerging area is the development of flexible and biocompatible microdevices for medical applications, such as implantable sensors and drug delivery systems. A case study of a research group developing a flexible biosensor for continuous glucose monitoring highlights the growing interest in flexible electronics. This trend has significant implications for the development of wearable medical devices and implantable sensors.
The exploration of new materials and processes continues to be a key driver of innovation in microfabrication. The development of novel materials with enhanced properties, such as improved strength, conductivity, or biocompatibility, is expanding the range of applications for microfabricated devices. For instance, the exploration of two-dimensional materials, such as graphene, is opening up new possibilities for advanced electronic and optical devices. Another emerging trend is the development of sustainable and environmentally friendly microfabrication processes. These processes aim to minimize waste and reduce the environmental impact of microfabrication. A case study of a research effort focused on developing environmentally friendly etching solutions shows the importance of sustainability in the microfabrication field. This trend reflects the growing awareness of environmental concerns and the need for sustainable technologies.
Conclusion
Microfabrication is a remarkable technology with immense potential to transform numerous industries. However, its journey from concept to commercial reality is fraught with challenges. From mastering the intricacies of microscale precision and navigating the complexities of cost-effective production, to bridging the gap between laboratory prototypes and market-ready products and embracing emerging trends, there are many obstacles to overcome. The journey requires innovation, collaboration, and a comprehensive understanding of both the technological and business aspects of this field. Overcoming these challenges will unlock the full potential of microfabrication and pave the way for even more groundbreaking advancements in the future.