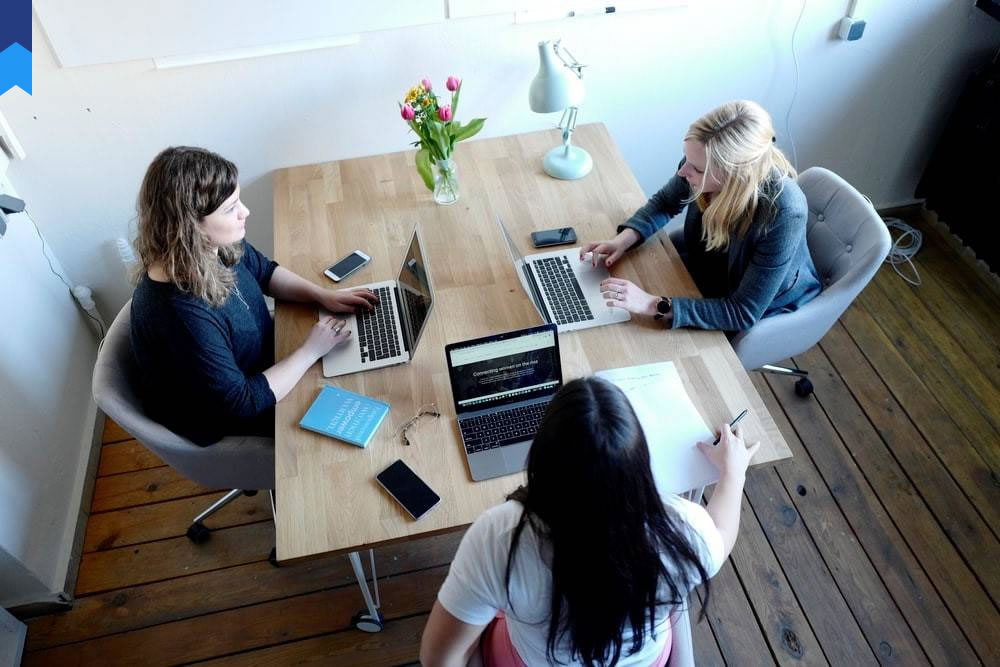
The Science Behind Hyper-Efficient Refrigeration
Refrigeration: A Deep Dive into the Science of Cooling
From preserving food to powering modern data centers, refrigeration is integral to our lives. Yet, its underlying science remains largely unappreciated. This article explores the unexpected and fascinating aspects of refrigeration technology, pushing beyond basic explanations to uncover the innovations shaping the future of cooling.
The Thermodynamics of Cooling: A Journey Beyond the Basics
At its core, refrigeration revolves around the laws of thermodynamics. The transfer of heat from a colder space to a warmer one requires energy input, a fundamental principle underpinned by the second law of thermodynamics. Conventional refrigeration cycles use refrigerants, substances with specific thermodynamic properties, to achieve this transfer. These refrigerants undergo phase transitions—from liquid to gas and back—absorbing heat during evaporation and releasing it during condensation. This cycle is driven by compressors, expansion valves, and heat exchangers. However, the efficiency of this cycle is limited by the Carnot efficiency, a theoretical maximum that depends on the temperature difference between the cold and hot reservoirs. Improving efficiency thus hinges on minimizing this difference, utilizing more efficient compressors, and deploying more effective refrigerants. Case study 1: the evolution from R-12 to more environmentally friendly refrigerants demonstrates a constant push for higher efficiency and reduced environmental impact. Case study 2: Comparing vapor-compression cycles to absorption refrigeration systems showcases different thermodynamic approaches to achieving cooling.
The quest for enhanced efficiency extends beyond the core cycle. Innovative designs such as magnetic refrigeration, using magnetocaloric materials, and thermoacoustic refrigeration, exploiting sound waves to transfer heat, challenge conventional approaches and aim for significantly higher energy efficiency. For example, magnetic refrigeration systems promise superior efficiency compared to vapor-compression systems, particularly at lower temperatures, where they can approach the Carnot limit more closely. Moreover, the development of highly efficient heat exchangers is crucial for maximizing performance. Modern designs incorporate microchannel technology, increasing the surface area for heat transfer and reducing pressure drop, leading to significant gains in efficiency. Another area of ongoing research involves optimizing the refrigerant itself. Researchers are exploring novel refrigerants with lower global warming potentials and superior thermodynamic properties, striving for a balance between performance and environmental responsibility. A notable example is the growing interest in natural refrigerants, such as ammonia, CO2, and propane, which often possess better environmental profiles than synthetic refrigerants.
The integration of smart technologies further boosts efficiency. Advanced control systems, utilizing sensors and algorithms, dynamically adjust the refrigeration cycle based on real-time conditions. This precision control minimizes energy waste, further enhancing overall system efficiency. Examples include variable-speed compressors, which adjust their output to match the cooling demand, and intelligent defrost cycles, optimized to minimize energy consumption. Furthermore, the integration of machine learning algorithms allows for predictive maintenance, preventing equipment failures and ensuring uninterrupted cooling operations. This predictive approach is becoming increasingly important in critical applications such as data centers and food storage facilities. Proper insulation is another key element that contributes significantly to the efficiency of any cooling system; reducing heat infiltration lowers energy requirements and minimizes the work the refrigeration system must perform. Innovative insulation materials, such as vacuum insulated panels, are continuously being developed to improve energy efficiency further.
The challenge of achieving higher efficiency is not merely an issue of optimizing components. It encompasses a broader systems approach, considering factors such as load management and energy storage. By managing the cooling load effectively and implementing energy storage solutions, such as thermal energy storage systems, the overall energy demand can be optimized, reducing peak load demands and improving energy consumption profiles. Examples of this integrated approach can be seen in modern commercial refrigeration systems which leverage innovative energy management strategies to minimize their overall footprint. The efficiency improvements in refrigeration technology are not solely driven by component-level advancements. They are equally driven by innovative design considerations that minimize energy losses and maximize operational efficiency. The future of refrigeration lies in pushing these boundaries further, creating systems that are not only more efficient but also more sustainable and environmentally friendly.
Refrigerant Technology: A Revolution in Green Cooling
The choice of refrigerant profoundly influences the environmental impact and efficiency of a refrigeration system. Traditional refrigerants like chlorofluorocarbons (CFCs) and hydrochlorofluorocarbons (HCFCs) were phased out due to their ozone depletion potential. However, even the hydrofluorocarbons (HFCs) that replaced them possess high global warming potentials (GWPs), contributing to climate change. This has sparked a massive shift towards low-GWP refrigerants, with a renewed focus on natural refrigerants such as ammonia (NH3), carbon dioxide (CO2), propane (R290), and isobutane (R600a). Ammonia, for instance, is widely used in industrial refrigeration due to its high efficiency and zero ozone depletion potential. However, its toxicity requires careful handling and system design. CO2, a naturally occurring refrigerant, offers a compelling alternative, possessing a GWP of 1, but requires higher operating pressures, demanding specialized equipment and safety measures. Propane and isobutane, known for their high efficiency and low GWP, are increasingly popular in domestic and smaller commercial applications.
The transition to low-GWP refrigerants necessitates significant changes in system design and manufacturing. For example, CO2 systems require specialized compressors and heat exchangers capable of withstanding high pressures. This calls for advances in materials science and manufacturing processes. Case study 1: A study comparing the energy efficiency of CO2 and traditional HFC-based systems in supermarkets shows that, despite the higher operating pressures, CO2 systems can achieve similar or better energy efficiency under certain conditions. Case study 2: Analysis of the life-cycle environmental impact of various refrigerants demonstrates that natural refrigerants, while requiring specialized equipment, often have a lower overall carbon footprint when considering their full life cycle. The development of novel refrigerants that combine high efficiency with exceptionally low GWPs remains a crucial area of research. Researchers are actively exploring various chemical compounds to identify those with desirable thermodynamic properties and minimal environmental impact. The exploration of alternative refrigeration technologies is not just limited to changes in refrigerant choice. For example, magnetic refrigeration utilizes the magnetocaloric effect in certain materials to achieve cooling. This technology offers the potential for highly efficient and environmentally friendly refrigeration, but it requires advancements in material science and system design to become commercially viable. Another area of research focuses on adsorption refrigeration, which employs the adsorption and desorption of refrigerants on a solid adsorbent to achieve cooling. This technology utilizes low-grade heat sources, opening up possibilities for waste heat recovery and enhanced energy efficiency.
Furthermore, the shift towards low-GWP refrigerants requires new safety regulations and standards. These regulations are crucial for ensuring the safe handling and installation of these refrigerants. For instance, the stricter safety requirements associated with ammonia necessitate specialized training for technicians involved in handling and maintaining ammonia-based refrigeration systems. Training programs and certification schemes are critical to the safe implementation of these new refrigerants. The transition to low-GWP refrigerants is not just a technological challenge; it is also a regulatory and societal one. Raising public awareness of the environmental benefits of these refrigerants and educating consumers about their usage and maintenance are crucial steps in successfully phasing out high-GWP alternatives. The collaboration between researchers, policymakers, manufacturers, and end-users is essential to ensure a smooth transition to sustainable refrigeration technology. The effectiveness of regulations depends heavily on robust enforcement mechanisms, ensuring compliance across all sectors. This collective effort is indispensable for realizing the full environmental benefits of this technological shift.
Beyond refrigerants themselves, the entire refrigeration cycle can be optimized for sustainability. This includes improving the energy efficiency of compressors, heat exchangers, and other components. Moreover, exploring innovative techniques like waste heat recovery can significantly reduce the overall energy consumption of refrigeration systems. Case studies comparing the energy efficiency of different refrigeration system designs, considering the entire lifecycle, are essential to guide informed decisions and policy changes. The ongoing development and refinement of low-GWP refrigerants will continue to be shaped by international agreements and regulations aiming to mitigate climate change. These agreements play a crucial role in driving innovation and market transformation. By aligning incentives and creating clear regulatory frameworks, these initiatives accelerate the adoption of sustainable refrigeration technologies. The future of sustainable refrigeration will depend heavily on a continued focus on research and development, along with the collaborative efforts of industry, governments, and research institutions to address the technical and regulatory challenges involved.
Smart Refrigeration: The Rise of Connected Cooling
The integration of smart technologies is revolutionizing refrigeration systems. Sensors, actuators, and sophisticated control systems enable real-time monitoring of system performance, predictive maintenance, and energy optimization. Smart refrigeration systems leverage data analytics to identify inefficiencies, anticipate potential problems, and adjust operating parameters dynamically to minimize energy consumption. For example, sensors can monitor refrigerant pressure, temperature, and flow rate, detecting anomalies that might indicate leaks or other malfunctions. This early detection allows for timely intervention, preventing costly repairs and downtime. Predictive maintenance algorithms analyze historical data to forecast potential equipment failures, enabling proactive maintenance before problems arise. This reduces unscheduled downtime and improves system reliability. Case study 1: A large supermarket chain implemented a smart refrigeration system that reduced energy consumption by 15% by optimizing compressor operation based on real-time demand. Case study 2: A data center used smart monitoring to detect a refrigerant leak early, minimizing the environmental impact and preventing a major service interruption.
Cloud-based connectivity further enhances the capabilities of smart refrigeration systems. Remote monitoring allows technicians to diagnose problems remotely, reducing response times and minimizing service disruptions. This remote diagnostics capability is particularly beneficial for large-scale refrigeration systems distributed across multiple locations. Cloud platforms also enable data aggregation and analysis across many systems, providing valuable insights into overall performance and identifying areas for improvement. The data collected can be used to develop more efficient operational strategies and optimize maintenance schedules. The use of machine learning algorithms further enhances the capabilities of smart refrigeration systems. These algorithms can identify patterns and anomalies in system data that might not be apparent to human operators. This advanced analytics capability allows for proactive optimization of system operation and more accurate prediction of maintenance needs. For example, machine learning can be used to predict the optimal defrost cycle based on real-time conditions, minimizing energy consumption while maintaining system performance. Furthermore, the integration of artificial intelligence (AI) is beginning to transform the field of refrigeration. AI-powered systems can learn and adapt to changing conditions, continuously optimizing energy efficiency and system reliability. This continuous optimization is a key advantage of AI-powered refrigeration systems.
However, the implementation of smart refrigeration systems presents challenges. The initial investment costs can be significant, requiring careful consideration of the return on investment. Moreover, concerns about data security and privacy must be addressed to ensure the responsible use of collected data. Robust cybersecurity measures are crucial to prevent unauthorized access to system data and protect against cyberattacks. Data privacy concerns necessitate clear guidelines and regulations concerning the collection, storage, and use of system data. The integration of smart technologies also necessitates skilled technicians capable of installing, maintaining, and troubleshooting these complex systems. Training programs and certification schemes are essential to ensure a skilled workforce capable of supporting the growing adoption of smart refrigeration technologies. Despite these challenges, the benefits of smart refrigeration are undeniable. The enhanced energy efficiency, reduced maintenance costs, and improved system reliability justify the investment in these technologies. The future of refrigeration will be increasingly driven by the integration of smart technologies, ushering in an era of efficient, reliable, and sustainable cooling solutions.
The convergence of smart technologies with environmentally friendly refrigerants is shaping the future of refrigeration. This combination promises both high efficiency and reduced environmental impact. For example, using smart sensors and control systems to optimize the operation of a CO2 refrigeration system can further enhance its energy efficiency and reduce its overall environmental footprint. The integration of smart technologies also facilitates the transition to novel refrigeration technologies, such as magnetic or thermoacoustic refrigeration, by providing tools for real-time monitoring and optimization. Smart refrigeration systems can monitor and control these more complex systems more effectively, enabling a smoother transition to these next-generation technologies. The combination of smart technology and sustainable refrigerants enables a holistic approach to sustainable cooling, addressing both energy efficiency and environmental concerns. By integrating smart sensors, controllers, and advanced algorithms, future refrigeration systems will be able to optimize their performance continuously, adapting to changing conditions and maximizing their efficiency and sustainability. This holistic approach is essential for achieving truly sustainable cooling solutions.
Refrigeration in a Changing Climate: Adapting to New Realities
Climate change is altering the landscape of refrigeration, demanding more efficient and sustainable solutions. Rising global temperatures increase the cooling demand, putting pressure on energy grids and exacerbating greenhouse gas emissions. Moreover, extreme weather events pose challenges to the reliability and safety of refrigeration systems, requiring robust designs capable of withstanding extreme temperatures and other environmental stresses. The need for greater energy efficiency is paramount. This necessitates innovation in refrigerant technology, system design, and operational strategies. Case study 1: A study on the impact of climate change on cooling demand shows that the increase in global temperatures will significantly amplify the energy demands for refrigeration. Case study 2: An analysis of the vulnerability of cold chain logistics to extreme weather events highlights the need for robust and resilient refrigeration systems in supply chain management.
Adapting to climate change requires a holistic approach, encompassing not just technological advancements but also policy interventions and societal changes. Stringent regulations and incentives for energy-efficient technologies are essential to accelerate the adoption of sustainable solutions. Moreover, education and awareness campaigns can promote responsible energy consumption and the use of sustainable cooling technologies. The development of robust infrastructure is also crucial, ensuring that the electric grid can handle the increasing cooling demand. This includes investing in renewable energy sources to decarbonize the energy supply for refrigeration systems. Smart grids and energy storage technologies can play a crucial role in managing the fluctuating demand for cooling, smoothing the load on power grids and optimizing the use of renewable energy sources. Improving insulation standards for buildings and transportation vehicles can significantly reduce the cooling load, minimizing energy consumption and the environmental impact of refrigeration. The adoption of passive cooling techniques, where natural processes are used to regulate temperature, can be a valuable supplementary strategy, reducing reliance on active cooling systems.
The development of resilient refrigeration systems is crucial for ensuring food security and public health in the face of climate change. These systems must be capable of withstanding extreme weather events and maintaining reliable cooling even during power outages. This necessitates robust designs and technologies, such as backup power systems, ensuring uninterrupted cooling in critical applications. Investing in resilient refrigeration infrastructure is crucial for protecting vulnerable populations and ensuring food security, especially in developing countries where climate change impacts are often most severe. Furthermore, the adoption of sustainable cooling practices extends beyond technology. It requires a broader societal shift towards conscious energy consumption, efficient food management, and minimizing waste generation. Implementing efficient food handling and storage practices can reduce spoilage and minimize the need for large-scale refrigeration. Education and awareness campaigns can encourage responsible behavior and promote the adoption of sustainable cooling practices across the population. The future of refrigeration hinges on a holistic approach that integrates technological advancements with policy interventions and societal changes, addressing both the immediate energy efficiency needs and the long-term impacts of climate change.
The increasing demand for cooling, driven by population growth and rising living standards, presents a significant challenge. Meeting this demand sustainably requires innovative solutions that minimize environmental impact while ensuring access to affordable cooling for all. This necessitates a multifaceted approach, encompassing technological innovations, policy interventions, and societal shifts. International collaboration is critical for addressing this global challenge effectively, sharing best practices, and promoting the adoption of sustainable cooling technologies across regions. Technological advancements in refrigeration, combined with policy initiatives such as carbon pricing and energy efficiency standards, can drive a global transition to more sustainable cooling solutions. The collaboration between governments, industries, and research institutions is paramount for successfully navigating this challenge and ensuring a sustainable future for refrigeration technology. The global community must work together to ensure that access to cooling is balanced with environmental responsibility, meeting the needs of a growing population without compromising the planet's health. This collective effort is essential for ensuring a sustainable future for cooling and safeguarding the well-being of future generations.
Conclusion
The science of refrigeration extends far beyond basic principles. Innovations in thermodynamics, refrigerant technology, smart systems, and climate adaptation are reshaping the field. The transition to low-GWP refrigerants, the integration of smart technologies, and the development of resilient systems are crucial steps towards a sustainable future. By embracing innovation, fostering collaboration, and implementing effective policies, we can ensure a future where cooling is efficient, reliable, and environmentally responsible. The journey toward hyper-efficient, sustainable refrigeration is an ongoing process, requiring continuous research, development, and collaboration across industries and nations. The challenge is not just technological, but also societal, demanding a shift in consumption patterns and a commitment to environmental stewardship. The future of refrigeration is not merely about technological prowess; it is about responsible innovation that balances human needs with environmental sustainability.