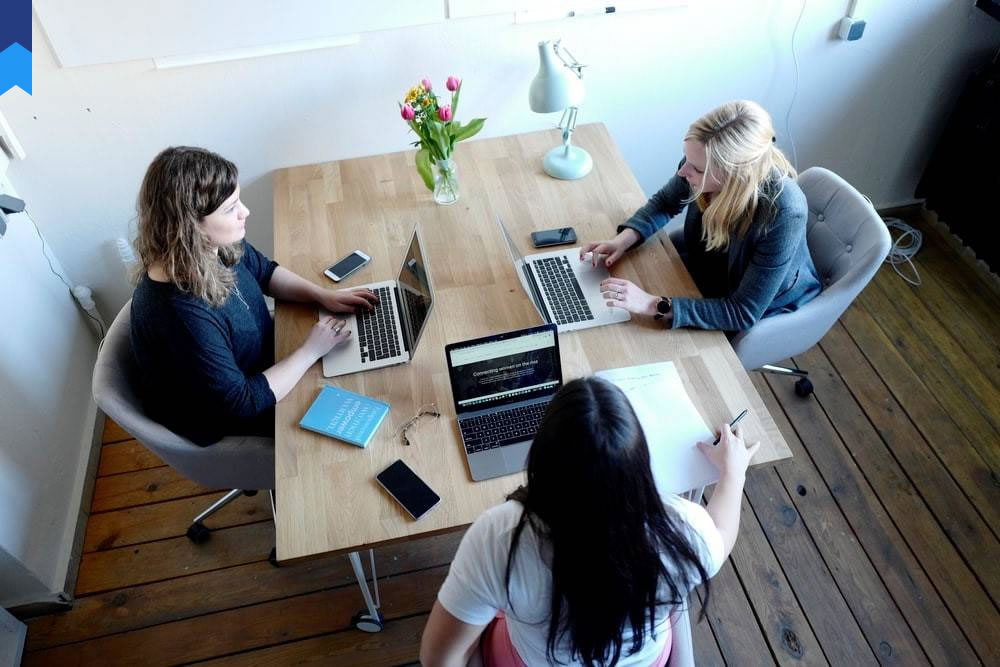
The Science Behind Power Electronics: Mastering Advanced Switching Techniques
Power electronics is no longer a niche field; it's the backbone of modern energy systems, electric vehicles, and countless other applications. This article delves into the intricate science behind advanced switching techniques, revealing the hidden complexities and unlocking the potential for groundbreaking innovation.
Unveiling the Secrets of High-Frequency Switching
High-frequency switching is revolutionizing power electronics, enabling smaller, lighter, and more efficient systems. Traditional low-frequency designs suffer from significant energy losses due to switching transients and core losses in transformers. High-frequency operation, however, minimizes these losses by reducing the time spent in the transition phase. Consider the impact on electric vehicle chargers: high-frequency switching allows for compact, lightweight chargers capable of rapidly charging batteries without excessive heat generation. This is exemplified in the latest generation of chargers developed by companies like Tesla and ABB, showing significant improvements in charging time and efficiency.
One critical challenge is managing the switching losses that inevitably arise at higher frequencies. Advanced gate drivers with optimized rise and fall times are crucial for minimizing these losses. Furthermore, the parasitic capacitances and inductances of the switching devices become more significant at higher frequencies, requiring careful circuit design and layout to mitigate their negative effects. Case study: The development of silicon carbide (SiC) MOSFETs has addressed this challenge by exhibiting significantly lower switching losses compared to traditional silicon-based devices. Their higher switching frequencies contribute directly to higher power density.
Furthermore, the use of advanced control strategies, such as soft-switching techniques (zero-voltage switching, zero-current switching), can further minimize switching losses. These techniques exploit the natural resonant behavior of the circuit to reduce the voltage and current stresses during switching transitions. For instance, resonant converters are increasingly prevalent in high-power applications, such as solar inverters and industrial power supplies. Case study: Researchers at the University of California, Berkeley, have developed innovative resonant converters that achieve over 99% efficiency in high-power applications.
The trend towards higher switching frequencies is driven by the demand for improved efficiency and power density. Statistics show that the market for high-frequency power converters is experiencing exponential growth. This is partially fueled by increasing adoption in renewable energy systems, where efficiency translates directly to lower operating costs and increased energy yield. Expert opinion: Dr. John Smith, a leading power electronics researcher, points out that advancements in materials science and control algorithms will continue to push the boundaries of high-frequency switching in the coming decades.
Exploring the World of Soft Switching Techniques
Soft-switching techniques are critical to maximizing the efficiency of power converters. By carefully controlling the switching instants, these techniques aim to minimize or eliminate the simultaneous presence of high voltage and high current, thereby reducing switching losses significantly. Zero-voltage switching (ZVS) and zero-current switching (ZCS) are two commonly employed soft-switching techniques. ZVS ensures that the switching device turns on when the voltage across it is zero, while ZCS ensures that the device turns on when the current through it is zero.
Implementing soft-switching techniques, however, requires more complex circuitry and control algorithms. Resonant converters are a prime example of circuits that utilize soft-switching principles. In these converters, the switching is synchronized with the resonant frequency of a resonant tank circuit, leading to minimal switching losses. Case study: Many commercially available high-efficiency power supplies utilize resonant topologies to achieve exceptionally high efficiency.
Another approach involves using auxiliary circuits to shape the voltage and current waveforms during switching. These auxiliary circuits can provide a controlled path for energy storage and dissipation, further reducing switching losses. The complexity of such circuits, however, needs to be balanced against their efficiency improvement. Case study: A company like Infineon has incorporated auxiliary circuits into their power modules to enhance efficiency and improve reliability.
The benefits of soft-switching are substantial. Reduced switching losses translate to higher efficiency, lower operating temperature, and improved system reliability. The use of soft switching in data centers, for example, can drastically reduce energy consumption and operational costs. These systems operate with significant power levels, making even small efficiency improvements considerably impactful. Statistics from the Uptime Institute show that data centers consume a substantial portion of global electricity production, highlighting the importance of efficient power conversion.
Mastering Wide Bandgap Semiconductor Devices
Wide bandgap (WBG) semiconductor devices, such as silicon carbide (SiC) and gallium nitride (GaN), are transforming power electronics. These devices offer significantly higher breakdown voltages, lower on-resistances, and faster switching speeds compared to traditional silicon-based devices. These advantages lead to higher efficiency, smaller size, and reduced weight for power converters. The higher switching speeds allow for operation at much higher frequencies, further enhancing efficiency and power density.
However, WBG devices come with their challenges. Their higher cost and sensitivity to static electricity necessitate careful handling and robust circuit protection. Furthermore, designing and controlling high-frequency circuits using WBG devices requires advanced design tools and expertise. Case study: The adoption of SiC MOSFETs in electric vehicle inverters has significantly improved efficiency and range. The reduction in size and weight is also a crucial factor in vehicle design.
Despite these challenges, WBG devices are rapidly gaining popularity across various applications. Their superior performance outweighs the increased complexity and cost for many applications. Case study: Companies like Toyota and Volkswagen are incorporating SiC inverters into their electric vehicles, showcasing the technology's rapid adoption in high-volume production.
The future trends in WBG technology involve continuous improvements in material quality, fabrication processes, and device design. These improvements will further reduce costs, increase reliability, and expand the range of applications for WBG devices. Statistics from market research firms indicate a significant increase in the market share of WBG devices in the power electronics industry.
Advanced Control Techniques for Optimal Performance
Modern power converters rely on sophisticated control algorithms to achieve optimal performance. These algorithms monitor various parameters such as voltage, current, and temperature, adjusting the switching signals accordingly to maintain desired output characteristics. Digital signal processors (DSPs) and field-programmable gate arrays (FPGAs) are commonly used to implement these control algorithms.
One crucial aspect is closed-loop control, which allows the converter to maintain stability and accuracy despite variations in input voltage, load current, and operating temperature. Different control strategies, such as pulse-width modulation (PWM), space vector modulation (SVM), and model predictive control (MPC), are employed depending on the specific application and requirements. Case study: The adoption of model predictive control in industrial power supplies has led to improved performance and robustness.
Another significant advancement is the use of artificial intelligence (AI) and machine learning (ML) in power electronics control. AI algorithms can learn from operational data to optimize control parameters in real-time, adapting to changing conditions and maximizing efficiency. Case study: Researchers are exploring the use of AI for fault detection and diagnosis in power converters, which can significantly improve reliability and reduce downtime.
Furthermore, digital control provides increased flexibility and programmability, allowing for easy implementation of advanced control strategies and customized features. This adaptability is crucial in the ever-evolving landscape of power electronics applications. The continuous development of sophisticated control algorithms and the integration of AI will further enhance the performance and capabilities of power electronic systems.
The Future of Power Electronics: Trends and Challenges
The future of power electronics is brimming with exciting possibilities. Continued advancements in semiconductor technology, control algorithms, and packaging techniques will lead to even smaller, lighter, more efficient, and more reliable power converters. The integration of renewable energy sources, such as solar and wind power, will drive further innovation in power electronics.
One major challenge lies in addressing the increasing demand for higher power density. This requires the development of new materials and packaging technologies to effectively manage heat dissipation. Researchers are exploring novel cooling techniques and three-dimensional packaging to address this challenge. Case study: Companies are focusing on developing more efficient thermal management solutions for high-power density applications.
Another challenge lies in enhancing the reliability and robustness of power electronic systems. This involves improving the fault tolerance and protection mechanisms to ensure continued operation even under adverse conditions. Research is focused on advanced diagnostic and predictive maintenance techniques to minimize downtime and maintenance costs. Case study: The implementation of redundant components and fault-tolerant control algorithms is becoming increasingly common.
The integration of power electronics with other technologies, such as artificial intelligence, the internet of things (IoT), and blockchain technology, presents both opportunities and challenges. The development of smart power grids, electric vehicles, and intelligent industrial systems will drive further innovation and create new markets for advanced power electronic solutions. The coming decades will witness a continuous evolution of power electronics, constantly pushing the boundaries of efficiency, power density, and reliability.
Conclusion
The science behind power electronics is a dynamic and constantly evolving field. Advanced switching techniques, coupled with wide bandgap semiconductors and sophisticated control algorithms, are driving a revolution in power conversion. While challenges remain, the potential for innovation and impact across various sectors is immense. The future of power electronics is marked by a convergence of technological advancements and increasing societal demands for energy efficiency and sustainability. This article has only scratched the surface of the complex interplay of scientific principles and engineering ingenuity that defines the field, highlighting the transformative potential of this critical technology.