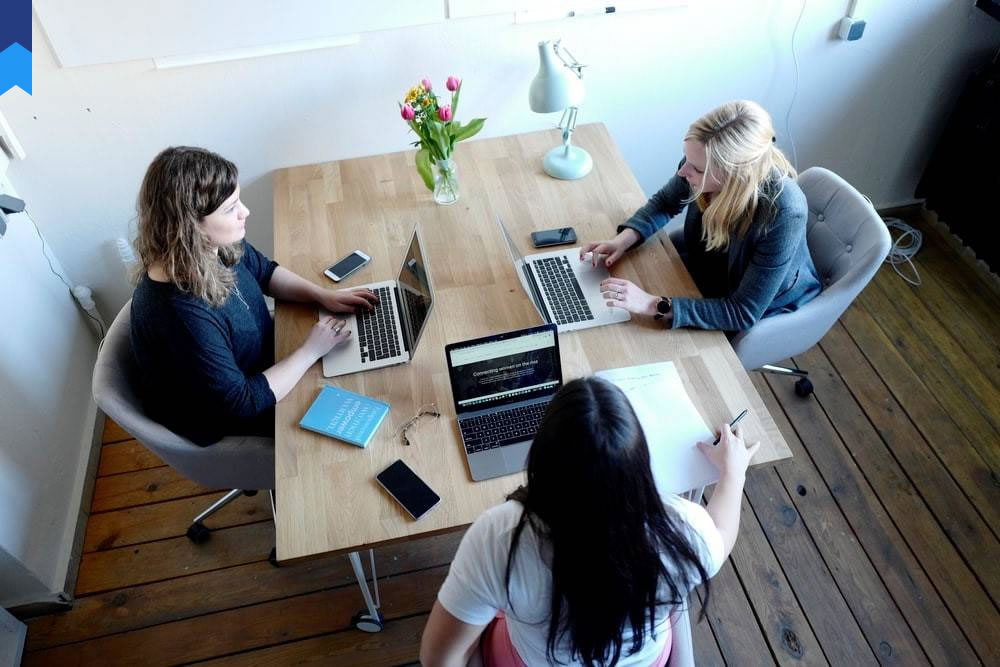
The Science Behind Solar Power Optimization
The sun's energy is a vast, untapped resource, promising a cleaner, more sustainable future. Harnessing this power effectively, however, requires a deep understanding of the science behind solar energy systems. This article delves into the complexities of solar power optimization, moving beyond basic overviews to explore innovative strategies and practical applications.
Maximizing Energy Harvest: Panel Placement and Angle
The efficiency of a solar energy system hinges critically on the strategic placement and precise angular orientation of solar panels. South-facing (Northern Hemisphere) installations generally maximize sunlight exposure. However, optimal angles vary based on latitude and seasonal changes in solar altitude. Detailed calculations, often aided by specialized software, are crucial for determining the ideal panel tilt and azimuth. Ignoring these factors can drastically reduce energy production.
Case Study 1: A residential installation in a high-latitude region saw a 20% increase in energy generation by adjusting panel angles to compensate for low winter sun angles. This involved using adjustable panel mounts that tracked the sun’s movement throughout the day.
Case Study 2: A commercial solar farm experienced a 15% efficiency boost after employing advanced algorithms to predict and adjust panel angles based on real-time weather conditions like cloud cover and atmospheric clarity. This dynamic adjustment significantly improved the energy capture.
Understanding the effects of shading is paramount. Even minor obstructions, like trees or neighboring buildings, can cast significant shadows on panels, reducing their output. Detailed shadow analysis, often using sophisticated 3D modeling software, is vital for maximizing energy yield. This includes accounting for changes in shadow patterns throughout the day and across different seasons.
The selection of appropriate panel technology is also crucial. Monocrystalline silicon panels generally boast higher efficiency than polycrystalline panels, though they tend to be more expensive. High-efficiency panels often have a higher upfront cost, but their superior energy generation can quickly offset the additional expense over the system's lifetime. Cost-benefit analyses should thoroughly consider long-term energy production compared to initial investment.
Careful consideration of panel spacing is essential to avoid shading between panels. This requires careful planning and design to ensure optimal placement and minimize inter-panel shading, improving the overall performance and energy output of the system. Moreover, choosing high quality inverters and wiring can minimize energy losses during conversion and transmission.
Effective energy harvesting also involves understanding the impact of weather conditions on solar panel performance. Dust, snow, and bird droppings can reduce energy output significantly. Regular cleaning and maintenance protocols are necessary to preserve system efficiency. Innovative cleaning solutions, such as self-cleaning panels with hydrophobic coatings, are becoming increasingly popular.
Advanced Energy Storage: Batteries and Beyond
Energy storage is crucial for maximizing the utilization of solar energy. Lithium-ion batteries remain the dominant technology for residential and small-scale commercial applications. However, their cost remains a barrier to widespread adoption, although prices are steadily declining. Research into alternative battery chemistries, such as solid-state batteries, is ongoing, aiming for higher energy density, improved safety, and longer lifespans. The potential for significantly reduced costs in the coming years makes alternative storage solutions compelling.
Case Study 1: A grid-tied solar system with battery storage enabled a homeowner to reduce their reliance on the electricity grid, significantly cutting energy bills while improving energy independence. The ability to store excess solar energy for nighttime use substantially decreased their dependence on the grid.
Case Study 2: A remote off-grid community successfully implemented a hybrid solar-wind energy system with advanced battery storage. This provided a reliable source of electricity in an area previously lacking access to a stable energy supply. The system’s robust energy storage capabilities addressed the intermittent nature of renewable resources, ensuring constant power availability.
Beyond batteries, other storage solutions are emerging. Thermal energy storage, for instance, uses solar heat to heat water or other materials, which can later be used for heating or other applications. This technology presents opportunities for efficient energy storage in contexts where direct electricity storage might be less practical.
Pumped hydro storage, a well-established technology, involves using excess electricity to pump water uphill, then releasing it to generate electricity when demand is high. This large-scale storage solution can be a vital component of integrating renewable energy sources into the wider power grid. Its scalability and proven reliability make it a key player in the energy transition.
The development of smart grids and advanced energy management systems enhances the efficiency of energy storage systems. Real-time monitoring and control allow for optimized charging and discharging of batteries, maximizing energy utilization and grid stability. Improved grid infrastructure and management are key to fully realizing the potential of solar energy storage.
Integrating different storage technologies, such as combining batteries with thermal or pumped hydro storage, can create more resilient and efficient energy systems. Hybrid approaches leverage the strengths of each technology to mitigate their individual limitations and offer a more balanced and robust solution.
Smart Grid Integration: Optimizing Energy Flow
The integration of solar energy systems into the smart grid is crucial for maximizing their impact. Smart grids utilize advanced sensors, communication networks, and data analytics to optimize energy flow and distribution. This allows for better integration of intermittent renewable energy sources, enhancing grid stability and reliability. The real-time data provided by smart grids allow for dynamic adjustments to energy generation and consumption, maximizing efficiency and minimizing waste.
Case Study 1: A municipality implemented a smart grid system to integrate a large number of residential solar systems. This enabled better management of electricity supply and demand, improving grid stability and reducing the need for peak power generation. The real-time data improved grid responsiveness to fluctuating renewable energy output.
Case Study 2: A large-scale solar farm integrated with a smart grid utilized advanced forecasting algorithms to predict energy output and adjust grid operations accordingly. This minimized disruptions and improved the reliability of the electricity supply, making it a more effective resource.
Demand-side management strategies, enabled by smart grid technologies, allow for better matching of energy supply and demand. This can include incentivizing consumers to shift energy consumption to off-peak hours, when solar generation may be lower. This flexible approach helps balance supply and demand, and it minimizes strain on the grid. Time-of-use pricing models also incentivize energy conservation and optimized consumption patterns.
Advanced metering infrastructure (AMI) provides detailed real-time data on energy consumption and generation, which facilitates improved grid management and energy optimization. This detailed information empowers utilities and consumers to make informed decisions about energy use and generation. AMI enables more precise forecasting and proactive grid management strategies.
The development of decentralized energy systems, where multiple smaller solar power generators are connected to the grid, enhances grid resilience and reliability. This distributed generation reduces reliance on large centralized power plants and improves the grid's ability to withstand disruptions. This approach ensures a more reliable energy distribution network, even during unforeseen events.
The integration of renewable energy sources into the grid also necessitates improved grid infrastructure to handle the variable nature of solar power. Upgrades to transmission lines, substations, and other grid components are essential for ensuring the safe and reliable integration of solar energy. This investment ensures a more resilient and adaptable power grid.
Innovative Technologies: Enhancing Efficiency
Continuous advancements in solar technology are constantly improving efficiency and reducing costs. Perovskite solar cells, for instance, offer the potential for significantly higher energy conversion efficiency compared to traditional silicon-based cells. While still in the early stages of commercialization, their potential for cost-effective high efficiency is considerable.
Case Study 1: A research team demonstrated a perovskite solar cell with over 25% efficiency, exceeding the performance of many silicon-based cells. This showcases the potential of perovskite technology to revolutionize solar energy generation.
Case Study 2: A company successfully integrated perovskite cells into a building-integrated photovoltaic (BIPV) system, demonstrating their potential for aesthetically pleasing and efficient solar energy solutions. This integration shows that perovskite cells can effectively generate power within architectural designs.
Concentrated solar power (CSP) systems use mirrors or lenses to concentrate sunlight onto a receiver, generating high-temperature heat that can be used to drive turbines or for other industrial processes. CSP offers the potential for large-scale energy production and thermal energy storage, extending energy availability beyond periods of direct sunlight. CSP is particularly suited for arid and sunny regions.
Advances in thin-film solar cells are also improving efficiency and flexibility. These cells can be manufactured on flexible substrates, allowing for their integration into various applications, such as clothing and building materials. This adaptability expands the scope of solar energy applications, making it a more versatile and pervasive technology.
The development of bifacial solar panels, which absorb light from both sides, further enhances energy production. These panels can capture reflected light from the ground, increasing their overall energy output compared to traditional panels. The additional energy generation from reflected light makes bifacial panels a more efficient option in specific environments.
Tracking systems, which automatically adjust the angle of solar panels to follow the sun throughout the day, maximize energy harvesting, particularly in areas with significant variations in solar altitude. These systems significantly improve energy capture, especially when paired with high-efficiency panels.
Policy and Regulation: Fostering Solar Growth
Supportive government policies and regulations are essential for accelerating the adoption of solar energy. Incentives such as tax credits, feed-in tariffs, and net metering programs can significantly reduce the cost of solar systems and encourage wider adoption. These financial incentives reduce the initial investment hurdle, making solar energy more accessible.
Case Study 1: A government's generous tax credit program for residential solar installations led to a dramatic increase in solar energy adoption, boosting the renewable energy sector and creating thousands of jobs. This demonstrates the effectiveness of financial incentives in stimulating the market.
Case Study 2: A region's implementation of a net metering program enabled homeowners with solar panels to sell excess electricity back to the grid, providing an added financial benefit and encouraging further investment in solar technology. This illustrates the potential of policy to create a positive feedback loop for solar adoption.
Streamlined permitting processes and reduced bureaucratic hurdles can accelerate the deployment of solar projects, minimizing delays and associated costs. These administrative improvements simplify the process of installing solar systems.
Investing in grid infrastructure and modernizing the electricity transmission system is necessary to accommodate the increasing integration of solar energy. Upgrading the grid to handle fluctuating renewable energy output reduces the risk of disruption. This enhances the reliability and stability of the power grid.
International cooperation and technology transfer can accelerate the global transition to solar energy. Sharing knowledge and resources across borders helps to optimize solar energy solutions and to improve global sustainability goals. This international collaboration promotes efficient and effective global deployment.
Promoting public awareness and education about the benefits of solar energy is crucial for encouraging wider adoption. This increased public understanding fosters greater acceptance and support for solar energy initiatives.
Conclusion
Optimizing solar energy systems requires a multi-faceted approach encompassing strategic panel placement, advanced energy storage, smart grid integration, innovative technologies, and supportive policies. By understanding the science behind these elements, we can unlock the full potential of solar energy and accelerate the transition to a cleaner, more sustainable future. The continued development and refinement of these aspects will be critical for achieving a truly sustainable and efficient energy landscape.