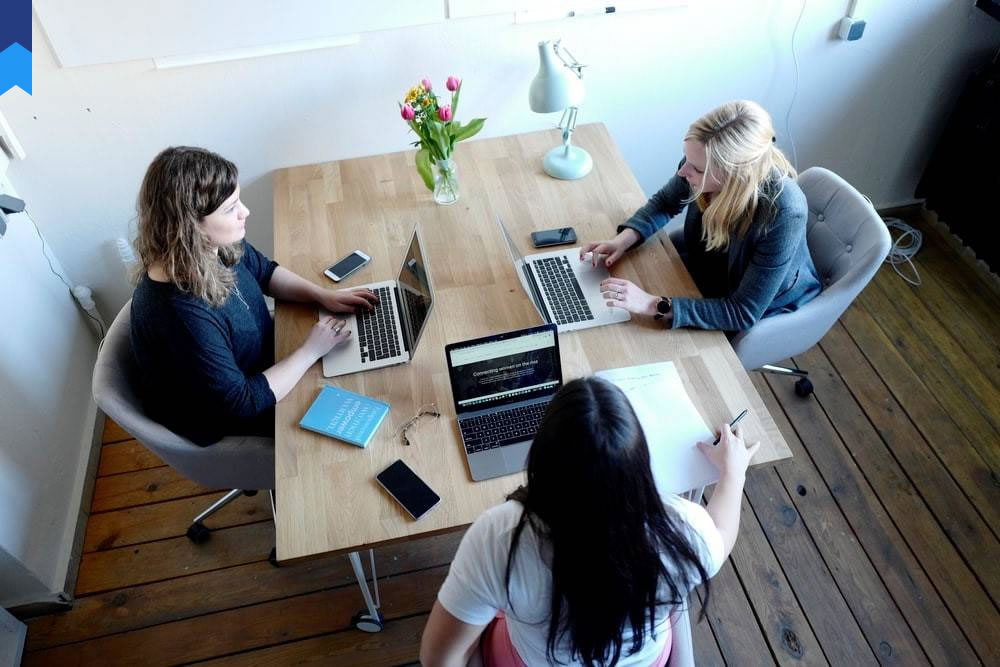
Uncovering The Truth About Antenna Design: Beyond The Basics
Antenna design is often viewed as a straightforward process, a simple extension of a communication system. However, a deeper dive reveals a complex interplay of physics, engineering, and material science, impacting everything from signal strength and efficiency to overall system performance. This article delves into the often-overlooked intricacies of antenna design, challenging conventional wisdom and presenting unexpected angles to illuminate the truth behind optimal antenna performance.
Understanding Polarization: More Than Just Vertical and Horizontal
Polarization, the orientation of the electric field in an electromagnetic wave, is a crucial element often simplified in basic antenna discussions. While vertical and horizontal polarizations are common, understanding the subtleties of circular and elliptical polarizations is key to optimizing signal reception, especially in challenging environments. Circular polarization, for example, offers resilience against signal fading caused by changes in the antenna's orientation relative to the transmitting source, making it ideal for satellite communication and mobile networks. Elliptical polarization, a hybrid, offers a compromise between the properties of linear and circular polarization, proving useful in various applications.
Consider the case of satellite television broadcasts. Many satellite dishes utilize circular polarization to ensure reliable reception even with slight misalignments. This is a critical advantage in consumer applications, minimizing the need for precise antenna alignment and enhancing the user experience. Similarly, in wireless communication systems, employing appropriate polarization techniques can minimize interference and improve signal quality, especially in dense urban environments. The use of orthogonal polarizations in cellular base stations, for example, allows for double the channel capacity in the same frequency band.
Further complicating matters is the fact that atmospheric conditions can impact polarization. Rain, snow, and even atmospheric turbulence can rotate or distort the polarization of the signal. The design of robust antenna systems needs to account for these effects, often through the use of polarization diversity techniques or specialized antenna designs. Understanding the practical implications of different polarizations and their susceptibility to environmental factors is essential for optimized system performance.
Moreover, advancements in antenna technology continually refine polarization capabilities. Research into metamaterials and advanced antenna arrays allows for dynamic control of polarization, which can be adapted to the changing environment or signal conditions. This enables adaptive antenna systems that self-optimize, further enhancing their performance and resilience. This adaptability is particularly crucial in dynamic environments like mobile networks, where signal conditions can vary significantly over short distances and time spans.
A case study in the development of high-performance antennas for 5G networks highlights the importance of polarization engineering. 5G systems require highly directional antennas with precise polarization control to maximize data rates and spectral efficiency. The design involved extensive simulation and testing to optimize polarization characteristics, ensuring reliable communication in a challenging radio frequency environment. Understanding the nuances of polarization proved vital for success.
The Impact of Antenna Size and Wavelength: A Misunderstood Relationship
The relationship between antenna size, wavelength, and performance is a fundamental principle of antenna design. However, it's frequently oversimplified. While it's true that larger antennas generally provide better directivity and gain, the actual relationship is far more nuanced. Antenna efficiency, for instance, is not solely determined by size, but also by the design's ability to effectively radiate energy. This efficiency is directly related to the antenna's impedance matching to the transmission line, a critical factor often overlooked in basic overviews.
Consider the case of microstrip antennas, which are commonly used in handheld devices. Their small size, relative to the operating wavelength, leads to lower gain and directivity. However, their compact form factor and ease of integration with circuit boards make them essential for these applications. On the other hand, large parabolic dish antennas, often used for satellite communication and radio astronomy, achieve exceptionally high gain and directivity thanks to their size, enabling them to receive weak signals from distant sources. The choice of antenna size is thus a trade-off between performance requirements and practical constraints.
Furthermore, advanced antenna design techniques, such as phased arrays and metamaterial-based antennas, can significantly alter this size-performance relationship. Phased arrays, for example, can electronically steer the beam, allowing for high gain and directivity even with relatively small individual antenna elements. Metamaterials, with their unique electromagnetic properties, allow for the miniaturization of antennas without compromising performance. This allows for design flexibility, especially important in applications requiring compactness.
A key case study involves the development of antennas for high-frequency radar systems. These systems demand high gain and directivity to detect and track targets at long ranges. Traditional designs would require massive and impractical antennas, making phased array technology a necessary alternative. The use of phased arrays enables the effective combination of multiple smaller elements, resulting in an overall performance matching that of a far larger antenna.
Similarly, in the field of nanoscale antennas, researchers are leveraging metamaterials and plasmonics to create extremely small antennas capable of operating at optical frequencies. These antennas hold significant promise for applications in optical communication and nanoscale sensing. The innovative design principles employed in these cases demonstrate a departure from the traditional relationship between size and performance, highlighting the ongoing evolution of antenna design.
The Role of Materials: Beyond Copper and Aluminum
Antenna performance is significantly influenced by the materials used in their construction. While copper and aluminum are widely used due to their excellent conductivity, modern antenna design increasingly incorporates advanced materials to enhance performance and enable novel functionalities. High-temperature superconductors, for example, offer the potential for extremely low-loss antennas, particularly beneficial in high-power applications. Meanwhile, metamaterials offer the ability to manipulate electromagnetic waves in ways not possible with conventional materials, leading to compact and high-performance designs.
A prime example is the use of carbon nanotubes in antenna construction. Their high conductivity and mechanical strength provide the potential for flexible and durable antennas for wearable electronics and other flexible applications. This represents a significant advancement over traditional rigid antenna structures. Moreover, the integration of different materials within a single antenna design allows for optimization of specific aspects of performance, such as impedance matching or bandwidth. This tailored material selection results in significantly enhanced antenna characteristics compared to uniform designs.
Another illustrative example is the development of antennas using piezoelectric materials. These materials convert mechanical stress into electrical energy and vice-versa, making them suitable for energy harvesting and sensing applications. Integrating piezoelectric materials into antennas allows for self-powered antennas or antennas with integrated sensing capabilities. The versatility offered by these advanced materials enables the creation of innovative and multi-functional antenna systems beyond the traditional capabilities of copper and aluminum.
A compelling case study is the development of antennas for extreme environmental conditions. Antennas deployed in high-temperature environments, such as those used in aerospace applications, require materials capable of withstanding extreme heat and maintaining performance. The careful selection of high-temperature dielectric and conducting materials is crucial for ensuring reliable operation in such challenging environments. The need to ensure material stability at these temperatures often overshadows the need for simply high conductivity.
Similarly, the development of antennas for biomedical applications necessitates the use of biocompatible materials that are safe for implantation in the body. The materials used must be both biocompatible and possess suitable electromagnetic properties. This intersection of material science and biomedical engineering opens new avenues for innovative antenna designs in medical applications, including implantable sensors and therapeutic devices. The selection of appropriate materials is paramount for achieving both safe and functional devices.
Advanced Antenna Techniques: Beyond the Dipole
Moving beyond simple dipole and monopole antennas, modern antenna design incorporates advanced techniques such as phased arrays, metamaterials, and fractal antennas to achieve enhanced performance and functionalities. Phased arrays, through their ability to electronically steer the beam, allow for high-directivity and gain without the need for physically moving antenna elements. This adaptability proves particularly crucial in applications like radar, satellite communication, and wireless communication, where beam steering is essential for efficient operation.
A remarkable example is the use of phased arrays in radar systems. These arrays allow the radar to scan a wide area efficiently without mechanically rotating the antenna, leading to faster scan rates and increased accuracy. The electronically controlled beam steering enables the focusing of energy precisely on areas of interest, enhancing target detection and tracking capabilities. This technology offers a significant advancement over older mechanical scanning radar systems.
Metamaterials, with their artificially engineered electromagnetic properties, provide unprecedented control over electromagnetic wave propagation. They can be designed to achieve functionalities not possible with naturally occurring materials, such as negative refractive index and perfect lensing. This opens up the possibility of creating extremely compact antennas with high performance. Miniaturization of antennas is a critical factor in modern electronics, and metamaterials provide significant advancement towards that goal.
Furthermore, fractal antennas, based on self-similar geometric patterns, possess unique properties such as wide bandwidth and multi-band operation. Their self-similarity results in efficient radiation over a wide range of frequencies, making them suitable for applications requiring multi-frequency communication or sensing. This wide bandwidth capability simplifies designs in applications needing simultaneous operation at multiple frequencies.
A case study illustrating the use of these advanced techniques is the development of antennas for 5G and beyond cellular networks. These systems require high data rates and low latency, making advanced antenna designs essential for efficient operation. The use of massive MIMO (multiple-input multiple-output) antenna arrays, which leverage phased array technology, and metamaterials for improved efficiency and miniaturization, is integral for optimizing the performance and efficiency of 5G and beyond cellular networks. This ongoing evolution of antenna technology directly affects the speed and reliability of mobile networks.
Conclusion
Antenna design is a field of continuous evolution, driven by the increasing demands for higher performance, greater efficiency, and novel functionalities. Moving beyond the basic understanding of antennas, this article explores the intricate details that influence performance, highlighting the importance of polarization, antenna size, material selection, and advanced antenna techniques. The examples and case studies presented demonstrate the practical implications of these concepts and showcase the ongoing innovation in this critical area of communication and sensing technology. As technology progresses, the sophisticated understanding and application of these principles will continue to drive further advancements in antenna design, paving the way for more efficient and capable communication and sensing systems in various applications.