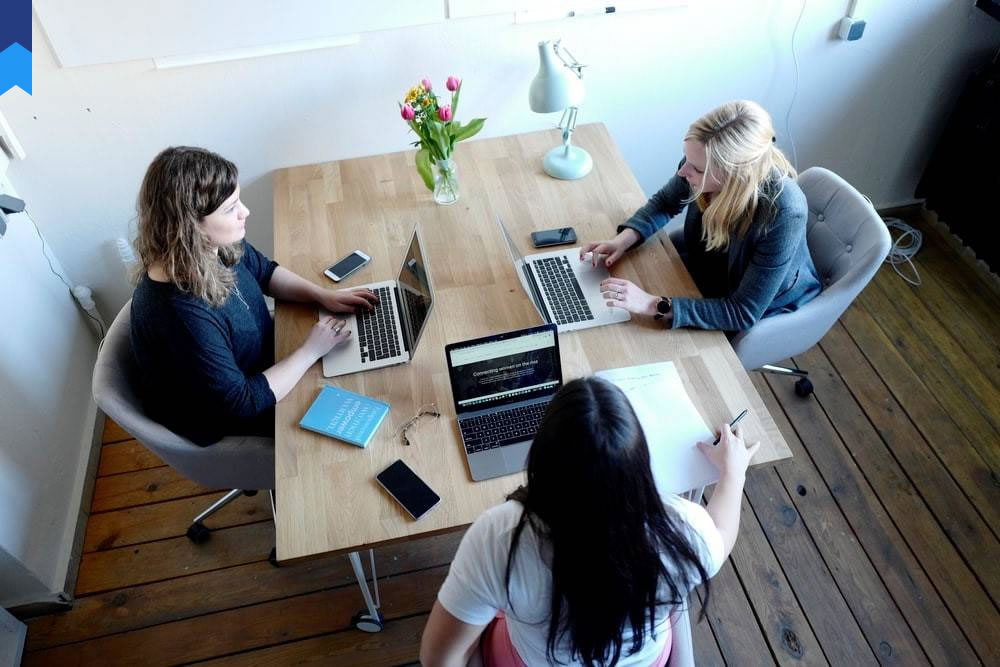
Uncovering The Truth About Smart Antennas
Smart antennas are revolutionizing the way we transmit and receive signals, going far beyond the basic functionality of traditional antennas. This article delves into the unexpected capabilities and often-overlooked realities of these sophisticated devices, challenging conventional wisdom and unveiling the complexities behind their seemingly simple design.
Adaptive Beamforming: Beyond Simple Signal Focusing
Adaptive beamforming is a cornerstone of smart antenna technology, enabling the dynamic shaping of transmitted signals to focus energy towards intended receivers while minimizing interference. Unlike traditional antennas with fixed beam patterns, smart antennas use multiple antenna elements and sophisticated signal processing algorithms to adjust their beam patterns in real-time. This adaptability is crucial in dynamic environments where obstacles or interference sources constantly change. Consider a cellular network in a bustling city: smart antennas can adapt to the changing positions of mobile devices and suppress interference from adjacent cell towers, resulting in improved signal quality and capacity. This dynamic adjustment is made possible through the use of sophisticated algorithms that analyze the incoming and outgoing signals and adjust the phase and amplitude of each antenna element. One notable case study is the implementation of adaptive beamforming in 5G networks, where it has been instrumental in achieving higher data rates and improved coverage. Another example is its use in wireless sensor networks for enhancing data transmission reliability in challenging environments with significant multipath fading.
Further advancements in adaptive beamforming involve exploring machine learning techniques to optimize the beamforming process. Machine learning algorithms can be trained on vast datasets of signal characteristics to predict optimal beam patterns in various scenarios. This approach enables more efficient resource allocation and improved performance in complex environments. For instance, research is underway to develop self-learning smart antennas that can autonomously adapt to unpredictable signal conditions, leading to more robust and efficient wireless communication systems. Furthermore, the integration of advanced signal processing techniques such as Multiple-Input and Multiple-Output (MIMO) with adaptive beamforming has created high-performance antennas that can simultaneously transmit and receive multiple data streams, significantly increasing network throughput. Practical implementations show a significant increase in data rates compared to traditional antenna systems. The ability of smart antennas to focus energy in a desired direction also reduces the potential for signal leakage, enhancing security and preventing eavesdropping.
The challenges involved in implementing adaptive beamforming include the computational complexity of the signal processing algorithms and the need for precise calibration of the antenna array. However, ongoing research and technological advancements are continuously improving the efficiency and robustness of these algorithms. Furthermore, the miniaturization of hardware components is making it feasible to integrate smart antennas into a wider range of devices, paving the way for the pervasive deployment of this technology. The increasing availability of high-performance processors and advanced digital signal processing chips has enabled the development of cost-effective solutions for smart antennas, making them more accessible for various applications.
Beyond cellular networks, adaptive beamforming finds applications in radar systems, satellite communication, and wireless sensor networks, improving detection accuracy, data throughput, and communication range. In radar systems, for example, adaptive beamforming can enhance target detection in cluttered environments by focusing the radar beam towards specific targets while suppressing clutter signals. In satellite communication, it can improve the link budget and reduce interference from adjacent satellites. The widespread adoption of these advanced technologies demonstrates the significant impact of adaptive beamforming on various domains. Future trends suggest an increasing integration of artificial intelligence and machine learning in beamforming algorithms, promising even more adaptable and efficient wireless communication systems.
MIMO Technology: Expanding Wireless Capacity
Multiple-Input and Multiple-Output (MIMO) technology is another critical aspect of smart antennas. MIMO systems utilize multiple transmitting and receiving antennas to enhance data transmission rates and reliability. By employing spatial multiplexing, MIMO systems can transmit multiple data streams simultaneously over the same frequency band, significantly increasing the overall capacity of the wireless link. Imagine a highway with multiple lanes: MIMO is analogous to adding more lanes, enabling more cars (data packets) to travel concurrently. A case study showcasing the effectiveness of MIMO is the deployment of 4G and 5G cellular networks, where MIMO has played a crucial role in improving data rates and network capacity. The ability to transmit multiple data streams simultaneously significantly enhances the user experience and enables applications that require high bandwidth. Another compelling example is its application in Wi-Fi routers, where MIMO technology enables higher speeds and improved coverage, benefiting users in demanding environments.
The performance of MIMO systems heavily relies on the accurate estimation of the channel characteristics, which describe how signals propagate between the transmitter and receiver. Channel estimation techniques are crucial in ensuring the efficient use of spatial resources and minimizing interference. Advanced channel estimation algorithms are constantly being developed to improve the accuracy and efficiency of MIMO systems in various environments. These algorithms leverage signal processing techniques and machine learning to account for multipath fading and other channel impairments. Sophisticated algorithms analyze signal variations to accurately determine the channel's characteristics and optimize data transmission accordingly.
One significant challenge associated with MIMO systems is the increasing complexity of the hardware and signal processing algorithms. As the number of antennas increases, the computational requirements and cost of implementation also rise. However, ongoing research focuses on developing more efficient algorithms and hardware solutions to address these challenges. Advances in integrated circuit technology and signal processing have made MIMO more cost-effective and practical for various applications. The development of compact and low-power MIMO systems has enabled their integration into smaller devices, expanding their applications.
The integration of MIMO with other advanced antenna technologies, such as adaptive beamforming, further enhances the performance of smart antennas. The combination of these technologies allows for highly efficient and reliable wireless communication. For example, in high-speed train communication, the combination of MIMO and adaptive beamforming ensures uninterrupted data transmission even when the train is moving at high speeds. Another example is in satellite communications, where the combination improves signal quality and resilience against interference. This synergy leads to high-performance systems with enhanced spectral efficiency and improved reliability. Future trends in MIMO research include exploring the use of massive MIMO systems with hundreds or even thousands of antennas, promising exponential increases in network capacity and data rates. The use of AI for optimal antenna placement and power allocation will further enhance the performance of these systems.
Beam Steering: Directing Signals with Precision
Beam steering, a key function of smart antennas, allows the antenna array to direct its radiation pattern towards a specific direction. This ability is essential for applications requiring precise signal targeting, such as radar systems, satellite communication, and wireless sensor networks. Unlike traditional antennas with fixed beam patterns, smart antennas use phased array technology to electronically steer the beam without any mechanical movement, enabling rapid and precise beam direction adjustments. This dynamic control is crucial in tracking moving targets or adjusting to changing environmental conditions. Consider a radar system tracking a moving aircraft: beam steering enables the radar to maintain a lock-on target even as the aircraft changes its position. A case study illustrating the efficiency of beam steering is the application in satellite communication networks, where it enhances signal strength and reduces interference from other satellites, leading to reliable and high-throughput communication. Another compelling example is in wireless sensor networks, where beam steering allows sensors to communicate effectively over long distances even in the presence of obstacles.
The accuracy of beam steering depends on the precision of the phase control and the design of the antenna array. Advanced control algorithms and precise hardware are crucial for achieving accurate beam pointing and low sidelobe levels. This control ensures that the majority of the signal energy is directed towards the intended receiver, minimizing interference. Advanced control algorithms account for variations in the environment to maintain accurate beam steering. These sophisticated algorithms involve using real-time feedback and adaptive signal processing techniques. The use of machine learning for optimizing beam steering is also a promising research area. These algorithms can learn from previous experiences and adapt to changing conditions, optimizing the beam steering parameters in real-time.
The challenges in beam steering include achieving high resolution and low sidelobe levels, particularly in large antenna arrays. High resolution is essential for accurate target tracking or signal selection, while low sidelobe levels ensure minimal interference with neighboring signals or systems. Advanced antenna designs and signal processing techniques are continuously being developed to address these challenges. Advanced antenna designs with specific element configurations can minimize sidelobe levels, while signal processing techniques can be used to suppress unwanted signals.
Beam steering is not only limited to point-to-point communication; it also plays a significant role in multi-user scenarios, allowing the antenna to dynamically serve multiple users simultaneously. This ability is critical in modern wireless communication systems that need to support a large number of users. For instance, in cellular base stations, beam steering enables efficient resource allocation and high data rates for multiple users, optimizing network capacity and throughput. In high-density wireless networks, such as those found in stadiums or airports, beam steering ensures efficient and reliable communication among numerous users. The future of beam steering involves the development of even more sophisticated algorithms and hardware components that can handle higher frequencies and larger antenna arrays, creating more advanced wireless systems with enhanced capabilities. The integration of AI for optimizing beam patterns and resource allocation will lead to more efficient and adaptable wireless communication systems.
Interference Mitigation: Combating Signal Noise
In dense wireless environments, interference from other signals can significantly degrade the quality and reliability of communication. Smart antennas play a vital role in mitigating interference through various techniques, ensuring robust and reliable communication. One approach is spatial filtering, where the antenna array selectively attenuates signals from interfering sources while enhancing signals from the desired source. This filtering is achieved through sophisticated signal processing algorithms that analyze the spatial characteristics of incoming signals and adjust the antenna response accordingly. Consider a Wi-Fi network operating in a crowded office building: smart antennas can filter out interference from other Wi-Fi networks and other electronic devices, improving the performance of the desired network. A case study demonstrating the effectiveness of spatial filtering is its application in military communication systems, where it enhances signal reliability in high-interference environments. The ability to filter out unwanted signals is vital for reliable communication in such settings. Another example is the use of smart antennas in cellular networks to minimize interference from neighboring cells, maximizing overall network capacity.
Another interference mitigation technique is the use of adaptive nulling, which creates deep nulls in the antenna's radiation pattern towards specific interfering sources. This approach selectively blocks signals from undesirable directions while maintaining sensitivity to signals from the desired direction. This precise control ensures that interference is minimized while preserving the signal of interest. Adaptive nulling algorithms constantly monitor the interference environment and adjust the antenna's response in real-time, dynamically adapting to changing interference patterns. The ability to dynamically adapt to changes in the environment is vital for maintaining consistent communication in dynamic environments. Sophisticated algorithms are employed to analyze signal characteristics and determine the optimal nulling pattern. The utilization of machine learning is also gaining traction in adaptive nulling, allowing systems to learn and predict interference patterns, improving overall performance.
Challenges in interference mitigation include the complexity of signal processing algorithms and the need for accurate channel estimation. Real-time processing of large amounts of data is required for effective interference mitigation, demanding high computational power and memory resources. Advanced signal processing techniques and efficient algorithms are crucial for overcoming these challenges. Advances in digital signal processing and parallel processing architectures are enabling the development of more powerful and efficient interference mitigation algorithms.
The integration of interference mitigation techniques with other smart antenna functionalities, such as beamforming and beam steering, further enhances overall performance. The combination of these functionalities leads to highly robust and efficient wireless communication systems, capable of operating in extremely challenging environments. For example, in crowded urban areas, the combined use of adaptive nulling and beamforming enhances data rates and improves network coverage. In satellite communication, interference mitigation enhances the reliability and throughput of communication links. The future of interference mitigation involves exploring the use of more sophisticated algorithms, including machine learning, to improve the effectiveness and adaptability of these techniques, leading to advanced wireless communication systems that can operate reliably in even more challenging environments. The use of artificial intelligence and machine learning is crucial in optimizing interference mitigation strategies, enhancing overall system performance and reliability.
Polarization Diversity: Enhancing Signal Reception
Polarization diversity is a technique that leverages the polarization properties of electromagnetic waves to improve signal reception and reliability. Electromagnetic waves can be polarized in different ways, such as linear or circular polarization. By using antennas with different polarization characteristics, polarization diversity enables the reception of signals that might be otherwise lost due to polarization mismatch. This technique is particularly valuable in environments with multipath fading or other signal impairments. Consider a wireless communication link in an urban environment with many buildings and obstacles. Signals may experience different polarizations as they reflect and diffract off objects. Polarization diversity can help ensure reliable reception by capturing signals with different polarizations. A case study showcasing the efficacy of polarization diversity is its application in satellite communication, where it improves signal reception despite the varying polarization of signals arriving from the satellite. The ability to capture signals with different polarization characteristics enhances signal quality and reliability. Another compelling example is the use of polarization diversity in high-speed train communications, where it mitigates signal fading due to multipath propagation and Doppler effects. The ability to mitigate these effects ensures reliable communication despite changing environmental conditions.
Implementing polarization diversity involves using antennas with different polarization characteristics, such as vertical and horizontal linear polarization or right-hand and left-hand circular polarization. Signal processing techniques are then used to combine the signals received from each antenna, enhancing signal quality and reducing the impact of fading. Advanced signal processing algorithms are used to optimally combine the signals from different polarizations, maximizing signal quality and minimizing interference.
Challenges in implementing polarization diversity include the increased hardware complexity and cost associated with using multiple antennas with different polarization characteristics. The added cost of having multiple antennas needs to be balanced against the improved signal quality and reliability. However, advancements in antenna technology and integrated circuit manufacturing are making polarization diversity more cost-effective.
The integration of polarization diversity with other smart antenna techniques, such as beamforming and interference mitigation, further enhances overall system performance. Combining polarization diversity with other techniques can improve signal quality and reliability significantly. For instance, combining it with beamforming enables more efficient use of the available spectrum. In scenarios with multiple users and interference sources, the combination of polarization diversity and interference mitigation techniques optimizes signal quality and network throughput. Future trends in polarization diversity involve exploring the use of more advanced polarization states and innovative antenna designs to further enhance signal reception and robustness. The exploration of advanced polarization states and antenna designs is crucial for achieving even higher performance in challenging wireless communication environments.
Conclusion
Smart antennas are not just incremental improvements over traditional antennas; they represent a paradigm shift in wireless communication. Their ability to adapt to dynamic environments, mitigate interference, and precisely steer signals opens up new possibilities in various applications. From enhancing cellular networks to enabling high-precision radar systems, smart antennas are driving innovation across numerous industries. The continuous advancements in signal processing, antenna design, and machine learning are paving the way for even more sophisticated and capable smart antenna technologies. The future promises more efficient, reliable, and secure wireless communication, thanks to the ongoing evolution of this transformative technology. Understanding the intricacies and capabilities of smart antennas is crucial for harnessing their full potential and shaping the future of wireless communication. The integration of AI and machine learning will further revolutionize the field, leading to self-configuring and self-optimizing smart antenna systems. The ongoing research and development efforts promise a future of even more advanced and capable smart antenna technologies.