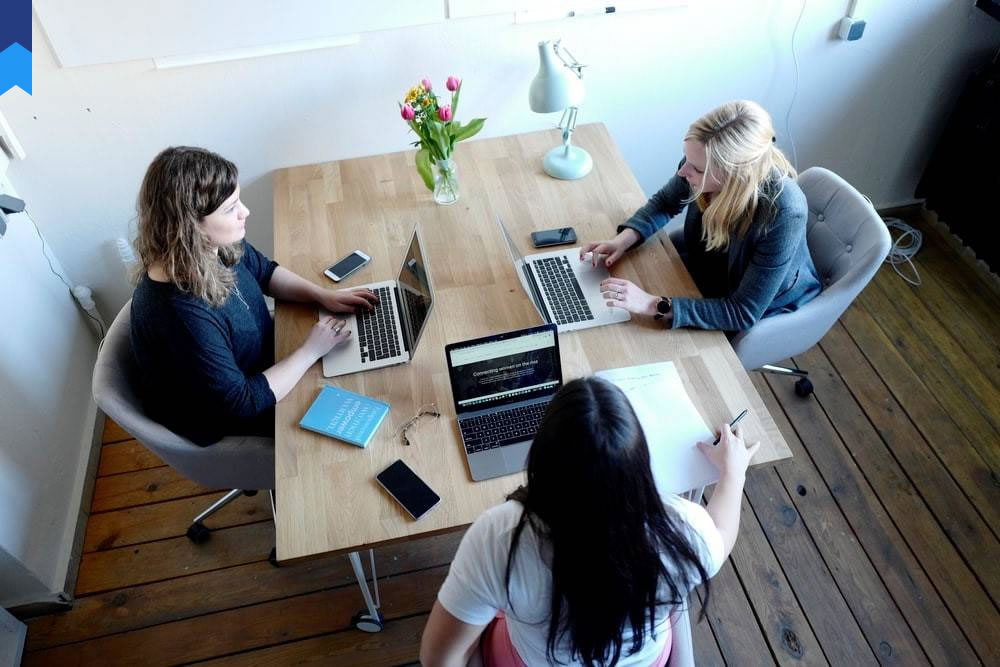
Unlocking The Secrets Of Geotechnical Site Characterization
Introduction
Engineering geology plays a crucial role in infrastructure development, ensuring the stability and longevity of structures. Geotechnical site characterization, a cornerstone of this discipline, involves a multifaceted approach to understanding subsurface conditions. This process dictates design parameters, construction methodologies, and ultimately, the safety and cost-effectiveness of any project. Accurate characterization prevents catastrophic failures and minimizes project delays and budget overruns. This article delves into the intricacies of advanced geotechnical site investigation techniques, exploring innovative approaches and best practices to provide a comprehensive understanding of this critical field.
Advanced Geophysical Techniques for Site Investigation
Traditional methods of site investigation, while essential, often offer limited spatial resolution. Advanced geophysical techniques, however, provide high-resolution subsurface imaging, allowing for a more precise understanding of soil stratigraphy and geological structures. Ground Penetrating Radar (GPR) is one such technique, offering detailed images of subsurface features and identifying potential hazards such as voids, fractures, and buried objects. GPR data can be processed to create three-dimensional models, significantly improving the accuracy of site characterization. For example, a recent project involving the construction of a high-speed rail line successfully utilized GPR to identify buried utilities and geological discontinuities, allowing engineers to design a foundation system that mitigated potential risks. Another case study involved the use of Electrical Resistivity Tomography (ERT), which measures the electrical conductivity of the subsurface to delineate different soil and rock units. ERT is particularly useful for detecting groundwater contamination and mapping the extent of contaminant plumes. The integration of GPR and ERT data provides a synergistic effect, resulting in a comprehensive subsurface model. Further enhancing geophysical data interpretation is the use of advanced processing and imaging techniques. Software advancements allow for improved noise reduction, better resolution, and the generation of 3D models, contributing to better decision-making.
Another significant advancement involves the use of seismic methods, such as seismic refraction and reflection surveys. These techniques utilize seismic waves to determine the velocity and geometry of subsurface layers, providing valuable information about soil and rock properties. Seismic refraction surveys are particularly useful for identifying the depth to bedrock and determining the thickness of overlying soil layers. Conversely, seismic reflection surveys provide a high-resolution image of the subsurface, allowing engineers to identify subtle geological structures that may impact the stability of a structure. A large dam construction project successfully utilized a combination of seismic techniques and borehole data to understand the complex geology of the site, enabling the design of a robust foundation system that resisted potential seismic events. The integration of multiple geophysical datasets provides a higher level of confidence in the subsurface model than any single technique alone. This multi-faceted approach greatly reduces the risk of errors and omissions that could result from relying on limited data.
Furthermore, the development of unmanned aerial vehicles (UAVs) equipped with high-resolution cameras and sensors offers new possibilities for site investigation. UAVs can efficiently survey large areas, providing high-resolution aerial imagery and orthomosaics. This data can be used to create detailed topographic models and identify surface features relevant to subsurface conditions. The integration of UAV-based data with other geotechnical data, such as geophysical surveys and borehole data, creates a more comprehensive and accurate subsurface model. A case study involving the development of a large-scale mining operation used UAVs to efficiently map the surface topography and identify potential geological hazards, aiding in the effective planning of mining operations. In another example, UAV-based LiDAR surveys proved crucial in precisely mapping the terrain for the construction of a major highway, optimizing the design and reducing construction costs. The ongoing evolution of UAV technology, including advancements in sensor capabilities and data processing techniques, continues to enhance the potential of this method for site investigation.
The advancements in data acquisition techniques are complemented by the development of sophisticated software for processing and interpreting geophysical data. Software packages now include advanced algorithms for noise reduction, image enhancement, and 3D modelling. This allows for a more efficient and accurate interpretation of geophysical data, reducing the reliance on subjective judgments. Experts use these tools to visualize complex subsurface structures and develop detailed geological models. The use of sophisticated software not only enhances the accuracy of the interpretation, but also provides a platform for collaboration and communication among engineers and geotechnical specialists. These advances enable faster and more accurate analyses, contributing to more informed decision-making during project design and execution. The future of geophysical site characterization will undoubtedly involve greater integration of these technologies, leading to more efficient and precise investigations.
In-situ Testing: Beyond the Standard Penetration Test
In-situ testing plays a critical role in determining the engineering properties of soil and rock. While the Standard Penetration Test (SPT) remains a widely used method, its limitations have driven the development of more advanced in-situ testing techniques offering greater precision and a wider range of information. Cone Penetration Testing (CPT) offers continuous measurement of soil resistance and pore water pressure, providing a detailed profile of soil stratigraphy and strength. CPT data is often used to correlate with laboratory testing results, providing a comprehensive understanding of soil behavior. A case study involving the design of a large-scale retaining wall successfully used CPT data to characterize the soil profile, leading to an optimized design that reduced the amount of earthwork required. In another instance, a deep excavation project relied heavily on CPT data to understand the soil’s liquefaction potential. The high-quality data allowed engineers to implement proper mitigation strategies, preventing potential failures.
Another advanced in-situ testing technique is the Pressuremeter Test (PMT), which measures the deformation characteristics of soil under controlled pressure. PMT provides valuable information on soil stiffness, strength, and stress-strain behavior. This data is particularly useful for the design of foundations and retaining structures in highly deformable soils. For example, a high-rise building project effectively utilized PMT data to optimize the design of the foundation system, ensuring stability on a particularly soft soil site. Another project involving the construction of an underground tunnel system in challenging geological conditions successfully employed PMT tests to determine the suitability of the proposed tunnel design. The PMT data provided valuable insight into the strength and deformation characteristics of the surrounding rock mass, ensuring the stability and safety of the tunnel.
Furthermore, seismic cone penetration testing (SCPT) combines CPT with seismic measurements to provide information on shear wave velocity, a key parameter for evaluating seismic hazard. SCPT data is useful for assessing the liquefaction potential of soils and designing foundations that are resistant to seismic events. A case study illustrating the value of SCPT was the design of a major bridge project in a seismically active region. SCPT provided detailed information on the shear wave velocity profile, allowing engineers to assess the liquefaction risk and design a foundation system to withstand potential earthquakes. In another application, SCPT was instrumental in evaluating the stability of an embankment dam in a seismically prone area. The data generated allowed engineers to implement mitigation strategies to reduce seismic risks and ensure the long-term stability of the dam.
The integration of multiple in-situ testing methods provides a more complete picture of subsurface conditions than any single technique alone. For instance, combining CPT and PMT data provides valuable insights into both the strength and deformation characteristics of soil, enabling a more refined assessment of foundation design parameters. Combining SPT and CPT data provides a powerful combination of traditional and modern testing methods, and this allows engineers to validate findings and optimize project decisions. Advanced data processing techniques and software now allow for the seamless integration of data from multiple in-situ testing methods, leading to a more comprehensive and accurate understanding of subsurface conditions. This data integration leads to higher accuracy and informed decision-making. The future of in-situ testing will undoubtedly include further integration of advanced sensors and data processing methods, leading to more efficient and effective site characterizations.
Laboratory Testing and Advanced Material Characterization
Laboratory testing remains a crucial component of geotechnical site characterization, providing detailed information on the physical and mechanical properties of soil and rock samples. While standard laboratory tests provide fundamental information, advanced techniques offer a more nuanced understanding of material behavior. For instance, advanced triaxial testing, employing sophisticated stress paths and measurement techniques, provides a more comprehensive assessment of soil strength and deformation characteristics under various loading conditions. A major highway project used advanced triaxial testing to determine the stability of a cut slope in challenging soil conditions, contributing to a successful and safe construction. In another project, the construction of a tall building necessitated extensive triaxial testing to evaluate the strength characteristics of the soil under expected loads, ensuring the building’s structural integrity.
Another sophisticated technique is resonant column testing, which measures the dynamic properties of soil, such as shear modulus and damping ratio. This information is critical for the design of structures subjected to dynamic loading, such as earthquakes and vibrations from nearby traffic. A significant case study demonstrating the importance of resonant column testing was the design of a nuclear power plant in a seismically active zone. The accurate determination of soil dynamic properties enabled the development of robust seismic design provisions, ensuring the plant's safety. Another instance showcased the use of resonant column testing for the design of a railway system close to sensitive structures. The analysis provided by this test ensured that vibrations wouldn’t have negative impacts on nearby buildings and infrastructure.
Furthermore, advanced imaging techniques, such as X-ray computed tomography (CT) scanning and scanning electron microscopy (SEM), allow for the detailed visualization of soil microstructure. This information provides insights into the fabric and pore structure of soils, enhancing understanding of their mechanical behavior. A research project investigating the performance of engineered soils used CT scanning to analyze the spatial distribution of particles in a compacted fill. The analysis yielded key insights into the effect of compaction parameters on soil strength and stability. In a similar vein, SEM analysis was critical in researching the behavior of expansive clays. The microscopy allowed researchers to visualize and understand the unique mineralogical composition and structure of these soils and to predict their behavior.
Integrating laboratory testing results with field data, such as in-situ testing and geophysical surveys, creates a robust and comprehensive geotechnical model. Advanced data analysis techniques and software allow for a more efficient integration of this data, improving the overall accuracy and reliability of site characterization. For instance, numerical modeling techniques and finite element analyses enable sophisticated analyses, considering complex geological conditions and loading scenarios. In essence, these combined methods provide a stronger prediction for the behavior of soils and thus allow for more robust design decisions. The future of laboratory testing will certainly involve further advancements in testing techniques and analytical methods, leading to more detailed and accurate characterization of geotechnical materials.
Numerical Modeling and Data Integration
Numerical modeling has become an indispensable tool in geotechnical engineering, enabling the simulation of complex geological conditions and loading scenarios. Finite element analysis (FEA) is a widely used technique that allows for the prediction of stress, strain, and displacement in soil and rock masses under various loading conditions. A major dam construction project successfully used FEA to model the stability of the dam under various hydrological and seismic loading conditions. The results of the simulations aided in the optimization of the dam's design, ensuring its stability and safety. A similar approach was used for the design of a large retaining wall near a riverbank, with FEA ensuring the stability under extreme hydrological loading conditions.
Another sophisticated modeling technique is discrete element modeling (DEM), which simulates the behavior of individual soil particles, providing a more detailed representation of soil mechanics. DEM is particularly useful for simulating the behavior of granular materials, such as sands and gravels, under various loading and shear conditions. A large-scale mining operation used DEM to simulate the stability of open-pit mine slopes, providing valuable insights into potential failure mechanisms. The analysis enabled engineers to implement mitigation strategies to reduce the risk of slope failures. Another application involved the use of DEM in simulating the behavior of granular materials during blasting operations in rock excavations, improving both safety and efficacy of the blasting processes.
Furthermore, the integration of multiple data sources, including in-situ testing, laboratory testing, and geophysical surveys, is crucial for creating a comprehensive and accurate geotechnical model. Advanced data integration techniques and software now allow for the seamless integration of data from diverse sources, leading to a more robust and reliable model. A case study involving the design of a complex underground infrastructure project successfully used integrated data from various sources to create a detailed three-dimensional model of the subsurface. This model accurately depicted subsurface conditions and allowed engineers to anticipate and mitigate potential risks during construction. Another major urban construction project involved the use of an integrated 3D model that incorporated geophysical data, borehole logs, and ground-penetrating radar results, providing critical information about the subsurface and optimizing construction planning.
The future of numerical modeling in geotechnical engineering will involve greater integration of advanced algorithms, machine learning, and artificial intelligence. These technologies will enable more efficient and accurate simulations of complex geological conditions and loading scenarios, providing valuable insights for improved design and construction practices. The use of high-performance computing resources will also enable the simulation of increasingly complex and large-scale geotechnical problems. This continuous technological advancement will lead to better understanding of complex subsurface conditions and optimize structural design even further.
Conclusion
Geotechnical site characterization is a dynamic field constantly evolving with technological advancements and improved understanding of soil and rock behavior. The integration of advanced geophysical techniques, in-situ testing, laboratory analyses, and numerical modeling creates a powerful framework for achieving a comprehensive and accurate understanding of subsurface conditions. This understanding is not just crucial for the design of safe and stable structures, but also for optimizing project costs and minimizing environmental impact. The future of this field lies in the continued development and integration of innovative technologies and approaches, driving further advancements in site characterization and enhancing the safety and reliability of infrastructure projects globally. The ongoing evolution of these techniques is crucial to ensuring safe and sustainable infrastructure development worldwide.