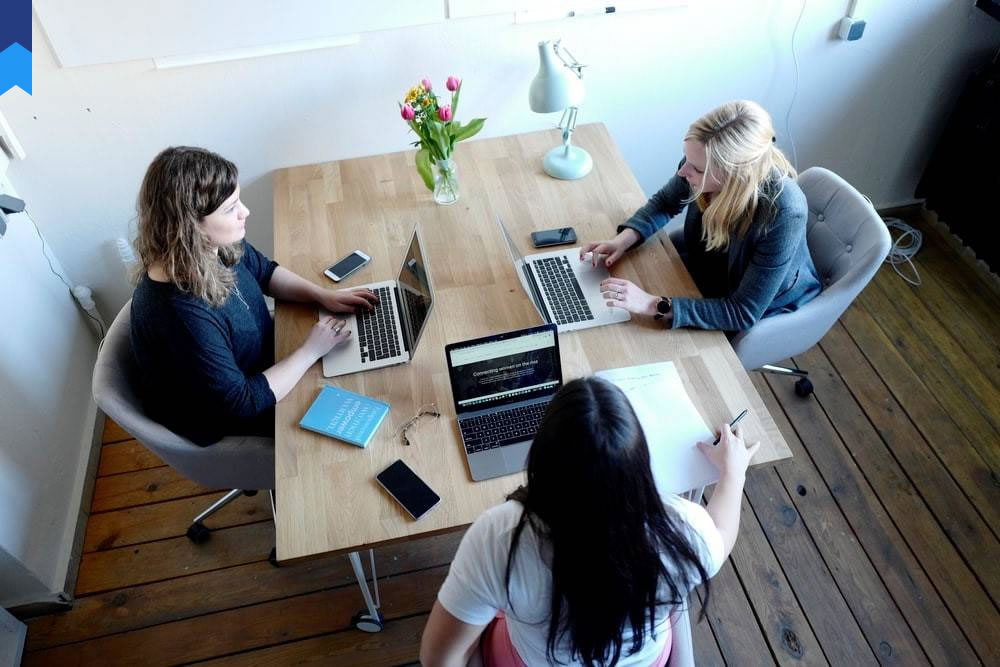
Unlocking The Secrets Of Quantum Computing
Quantum computing, a field once relegated to the realms of theoretical physics, is rapidly transitioning into a tangible technological force. Its potential to revolutionize industries from medicine to finance is immense, yet understanding its complexities remains a challenge. This article delves into the intricacies of this revolutionary technology, exploring its practical applications and unveiling the secrets behind its groundbreaking potential.
The Fundamental Principles of Quantum Computing
Unlike classical computers that store information as bits representing 0 or 1, quantum computers leverage quantum bits, or qubits. Qubits exploit quantum mechanical phenomena like superposition and entanglement to perform computations far beyond the capabilities of classical computers. Superposition allows a qubit to exist in a probabilistic state of both 0 and 1 simultaneously, while entanglement links two or more qubits, enabling them to share a combined state regardless of distance. This allows for vastly accelerated calculations on complex problems currently intractable for even the most powerful supercomputers. Consider the problem of factoring large numbers, a cornerstone of modern cryptography. Classical algorithms struggle with this, but Shor's algorithm, a quantum algorithm, offers a polynomial-time solution, posing a significant threat to current encryption standards. IBM's quantum computer, for instance, demonstrates the power of superposition through practical application in simulating molecular behavior, a feat impossible for classical computing at scale. Another example of practical implementation is Google's Sycamore processor, which achieved “quantum supremacy†by solving a specific problem exponentially faster than any classical computer. This showcases the potential of superposition to drastically reduce computation time for specific tasks.
Entanglement, another key principle, allows for correlations between qubits that transcend classical understanding. If two entangled qubits are measured, the outcome of one measurement instantly influences the outcome of the other, regardless of the physical separation between them. This phenomenon, seemingly paradoxical from a classical perspective, enables the creation of highly interconnected quantum systems capable of exploring a vast solution space simultaneously. The development of robust entanglement protocols and error correction techniques are paramount to realizing the full potential of quantum computers. For example, researchers at the University of Innsbruck have demonstrated highly entangled states of up to 20 ions, paving the way for larger, more complex quantum systems. This represents a crucial step towards building practical quantum computers capable of solving real-world problems. A further case study involves researchers at the University of Science and Technology of China who successfully teleported quantum information over a distance of 1,200 kilometers using entangled photons. Such long-distance entanglement is critical for building quantum networks and distributed quantum computing systems.
Quantum algorithms, designed to exploit the unique properties of quantum mechanics, are essential for unlocking the power of quantum computers. These algorithms are often fundamentally different from classical algorithms, leveraging superposition and entanglement to achieve exponential speedups for specific types of problems. Shor's algorithm, mentioned earlier, is a prime example, as is Grover's algorithm, which provides a quadratic speedup for unstructured search problems. While not all problems benefit from quantum acceleration, the existence of these algorithms underscores the transformative potential of this technology. The development of new quantum algorithms and the adaptation of existing classical algorithms to the quantum framework are ongoing areas of intensive research, with breakthroughs constantly being made.
The practical implementation of quantum computing relies on several physical platforms, each presenting unique advantages and challenges. Superconducting circuits, trapped ions, photonic systems, and neutral atoms are among the most promising technologies. Each platform faces distinct hurdles in terms of scalability, coherence time (the duration qubits maintain their quantum state), and error rates. Significant advancements are being made in these areas, with researchers constantly striving to improve the fidelity and scalability of quantum computing hardware. For instance, Google's approach using superconducting qubits shows significant progress in terms of scalability, while IonQ's trapped ion technology boasts high qubit fidelity. Comparing these different approaches is crucial for determining the most viable path towards fault-tolerant quantum computers. Another noteworthy example is the development of topological qubits, which are theoretically less susceptible to environmental noise and decoherence, representing a potential breakthrough in the quest for stable quantum computation. The ongoing exploration of various physical implementations highlights the collaborative and competitive nature of quantum computing research.
Quantum Computing Applications in Medicine
The potential of quantum computing to revolutionize drug discovery and development is immense. Traditional methods often rely on lengthy and expensive processes, but quantum algorithms can dramatically accelerate the process of identifying potential drug candidates and predicting their effectiveness. Quantum simulations can accurately model the behavior of molecules, enabling researchers to design drugs with greater precision. This is particularly useful in personalized medicine, where treatments can be tailored to individual patients based on their specific genetic makeup. A case study involves using quantum computing to simulate the behavior of proteins involved in diseases like Alzheimer’s, leading to the identification of potential drug targets that were previously unknown. Another example is the application of quantum computing to analyze large datasets of genomic information to identify genetic markers associated with diseases, enabling early detection and personalized treatment strategies. The use of quantum algorithms for analyzing medical images can also improve diagnostic accuracy and speed, contributing to faster and more effective medical interventions.
Quantum computing's role in developing new materials for medical applications is also significant. By simulating the interactions between atoms and molecules, quantum computers can help design new materials with enhanced properties, such as biocompatibility, strength, and durability. This allows for the development of improved implants, prosthetics, and drug delivery systems. For instance, quantum simulation can be used to design new biomaterials with improved osseointegration properties for orthopedic implants, leading to faster healing times and reduced complications. Another example lies in designing novel drug delivery systems that can target specific cells or tissues, improving the efficacy and reducing the side effects of medications. The ability of quantum computers to analyze vast amounts of data allows for the identification of patterns and relationships that are invisible to classical methods, accelerating the process of discovering new materials and improving existing ones.
Quantum machine learning, a subfield of quantum computing, is poised to transform medical image analysis. Quantum algorithms can be used to train machine learning models that can diagnose diseases from medical images with greater accuracy and speed than classical methods. This can lead to earlier detection of diseases and improved patient outcomes. A case study involves the use of quantum machine learning to analyze MRI scans to detect cancerous tumors with higher accuracy than traditional methods. Another example is the use of quantum algorithms to analyze electrocardiograms (ECGs) to detect heart conditions, allowing for timely interventions and improved patient management. These advancements show the potential of quantum computing to improve diagnostic capabilities and enhance the overall quality of healthcare.
Beyond drug discovery and materials science, quantum computing holds promise for enhancing various aspects of healthcare. This includes improving the accuracy and speed of medical diagnostics, enabling personalized medicine, and accelerating the development of new medical technologies. For example, quantum algorithms can be used to optimize the scheduling of medical appointments and resources, reducing waiting times and improving efficiency. Furthermore, quantum computing could improve the design of medical devices and improve the accuracy of radiation therapy treatments, minimizing side effects and maximizing therapeutic efficacy. The potential applications of quantum computing in healthcare are vast and continue to evolve, showcasing its transformative power.
Quantum Computing's Impact on Finance
The financial industry, with its reliance on complex data analysis and risk management, stands to greatly benefit from quantum computing. Quantum algorithms can significantly improve the speed and accuracy of portfolio optimization, fraud detection, and risk assessment. Quantum computers can analyze vast datasets of financial information, identifying patterns and correlations that are invisible to classical methods. This allows for more informed investment decisions and reduced financial risk. A case study involves the use of quantum algorithms to optimize investment portfolios, taking into account numerous factors and constraints, leading to improved returns and reduced volatility. Another example demonstrates the application of quantum computing to detect fraudulent transactions by identifying anomalies in large datasets of financial transactions, improving the efficiency and accuracy of fraud detection systems.
Quantum computing can revolutionize risk management by providing more accurate and efficient methods for assessing and mitigating financial risk. Classical methods often struggle to accurately model complex financial systems, but quantum algorithms can handle significantly larger and more complex datasets, enabling more accurate risk assessments. A case study highlights the use of quantum simulations to model the behavior of financial markets, enabling more accurate predictions of market trends and potential risks. Another example uses quantum computing to analyze credit risk more effectively, allowing financial institutions to make more informed lending decisions and reduce defaults. Quantum approaches offer significant advancements in assessing various financial risks such as credit risk, market risk, and operational risk, improving the stability and resilience of the financial system.
The potential for quantum computing to enhance high-frequency trading is also significant. Quantum algorithms can significantly accelerate the speed of execution and improve the efficiency of trading strategies. This allows for faster execution of trades and improved profitability. A case study describes the use of quantum algorithms to optimize trading strategies, enabling faster execution and improved returns. Another example focuses on using quantum computing to analyze real-time market data to make informed trading decisions, improving the performance of high-frequency trading algorithms. The speed and accuracy offered by quantum computing can be transformative for high-frequency trading, providing significant competitive advantages.
Quantum cryptography, a field leveraging the principles of quantum mechanics for secure communication, holds significant potential for securing financial transactions. Quantum key distribution (QKD) ensures the secure exchange of cryptographic keys, making it virtually impossible for eavesdroppers to intercept sensitive financial information. A case study involves the implementation of QKD in securing financial transactions between banks, ensuring the confidentiality of sensitive financial data. Another example highlights the use of quantum cryptography to protect against cyberattacks and data breaches, ensuring the security and integrity of financial systems. The use of quantum cryptography offers a significant enhancement in security, protecting sensitive financial data from increasingly sophisticated cyber threats.
Quantum Computing in Materials Science
Quantum computing offers unparalleled capabilities for simulating the behavior of materials at the atomic and molecular level. This allows researchers to design novel materials with tailored properties for various applications. Traditional methods often rely on approximations, but quantum computers can accurately model complex interactions, leading to the discovery of materials with enhanced strength, conductivity, or other desired characteristics. A case study involves using quantum simulations to discover new superconductors with higher critical temperatures, which could revolutionize energy transmission and storage. Another example highlights the application of quantum computing to design novel catalysts for chemical reactions, leading to more efficient and sustainable industrial processes.
The development of new materials with superior properties is crucial across numerous industries. Quantum simulations enable researchers to explore the vast chemical space, identifying materials with specific desired traits. This accelerates the discovery process and reduces the need for extensive experimental trials. A case study focuses on the design of new lightweight yet high-strength materials for aerospace applications, reducing fuel consumption and increasing efficiency. Another example uses quantum computing to develop new battery materials with higher energy densities and longer lifespans, addressing the growing demand for sustainable energy solutions. These advancements contribute to improved efficiency, sustainability, and economic benefits across diverse sectors.
Beyond the discovery of new materials, quantum computing offers the ability to optimize existing materials and manufacturing processes. By simulating the behavior of materials under various conditions, researchers can improve the design of existing materials and processes, enhancing their performance and efficiency. A case study describes the use of quantum computing to optimize the manufacturing process of semiconductors, leading to improved yield and reduced costs. Another example shows how quantum simulations can be used to improve the design of solar cells, enhancing their efficiency and reducing their manufacturing costs. These optimizations contribute to the improvement of existing technologies and the development of more cost-effective and sustainable manufacturing processes.
The ability to precisely model material properties at the atomic level allows for the targeted design of materials for specific applications, leading to innovative solutions in various fields. A case study involves using quantum computing to design new materials for quantum computing hardware itself, improving the coherence times and scalability of future quantum computers. Another example uses quantum simulations to design new biocompatible materials for medical implants, improving their integration with the human body and reducing the risk of rejection. The advancements in material science driven by quantum computing pave the way for breakthroughs in various technologies and contribute to improvements in quality of life.
Challenges and Future Trends in Quantum Computing
Despite the immense potential, several significant challenges hinder the widespread adoption of quantum computing. The primary obstacle is the inherent fragility of qubits, which are highly susceptible to decoherence—the loss of quantum information due to interactions with the environment. Maintaining the coherence of qubits for sufficiently long periods is crucial for performing complex computations. Significant advancements in error correction and qubit stabilization techniques are necessary to overcome this challenge. Research focuses on developing more robust qubits and implementing sophisticated error-correction codes to mitigate the effects of decoherence. Another crucial aspect is the need for improved quantum algorithms and software that can fully exploit the power of quantum hardware. Currently, the development of quantum algorithms lags behind the rapid progress in hardware development. Increased efforts are needed in algorithm design and software development to fully utilize the potential of quantum computers.
Scalability remains a major hurdle. Building quantum computers with a large number of qubits is essential for tackling complex real-world problems. Current quantum computers have a limited number of qubits, restricting their computational power. Advances in fabrication techniques and qubit architecture are needed to significantly increase the number of qubits while maintaining coherence and low error rates. Modular quantum computing architectures are being explored to address the scalability challenge, allowing for the interconnection of multiple smaller quantum processors. This approach aims to build larger, more powerful quantum computers by combining smaller, more manageable units. Another significant aspect is the development of efficient methods for controlling and manipulating a large number of qubits with high precision. This requires significant advancements in both hardware and software.
The development of fault-tolerant quantum computers is a crucial milestone for widespread adoption. Fault tolerance protects against errors that inevitably occur during quantum computations, ensuring reliable and accurate results. Developing robust error correction techniques is paramount for building fault-tolerant quantum computers. Significant advancements are being made in topological quantum computing, which theoretically offers inherent fault tolerance. The development of novel error-correction codes and fault-tolerant architectures is crucial for achieving this goal. Another approach involves the development of hybrid quantum-classical algorithms, where classical computers are used to handle certain aspects of the computation, reducing the reliance on fault-free quantum computation.
The future of quantum computing hinges on overcoming these challenges and fostering collaboration across academia and industry. Continuous research and development are essential for advancing the technology and bringing it closer to its full potential. Increased investment in quantum computing research and development, coupled with interdisciplinary collaboration, will accelerate progress towards building practical, scalable, and fault-tolerant quantum computers. The potential benefits of quantum computing are immense, and overcoming these challenges will unlock transformative advancements across numerous fields. Future trends suggest that the integration of quantum computing with other technologies, such as artificial intelligence and machine learning, will create new opportunities and further expand its capabilities. This interdisciplinary approach will accelerate innovation and foster the development of new applications.
Conclusion
Quantum computing, while still in its nascent stages, holds the promise of transforming numerous industries. Its ability to solve currently intractable problems, coupled with its potential to revolutionize fields like medicine, finance, and materials science, is truly transformative. While significant challenges remain, the ongoing research and development efforts worldwide demonstrate a strong commitment to unlocking the full potential of this revolutionary technology. The convergence of scientific breakthroughs, increased funding, and collaborative efforts are paving the way for a future where quantum computing becomes an indispensable tool, shaping the technological landscape in unprecedented ways. The future likely involves a gradual integration of quantum computing into existing technologies, enhancing current capabilities and opening up entirely new possibilities. As the technology matures, we can expect to see even more profound impacts across a wide range of applications, leading to a technological revolution on a par with the digital revolution itself.