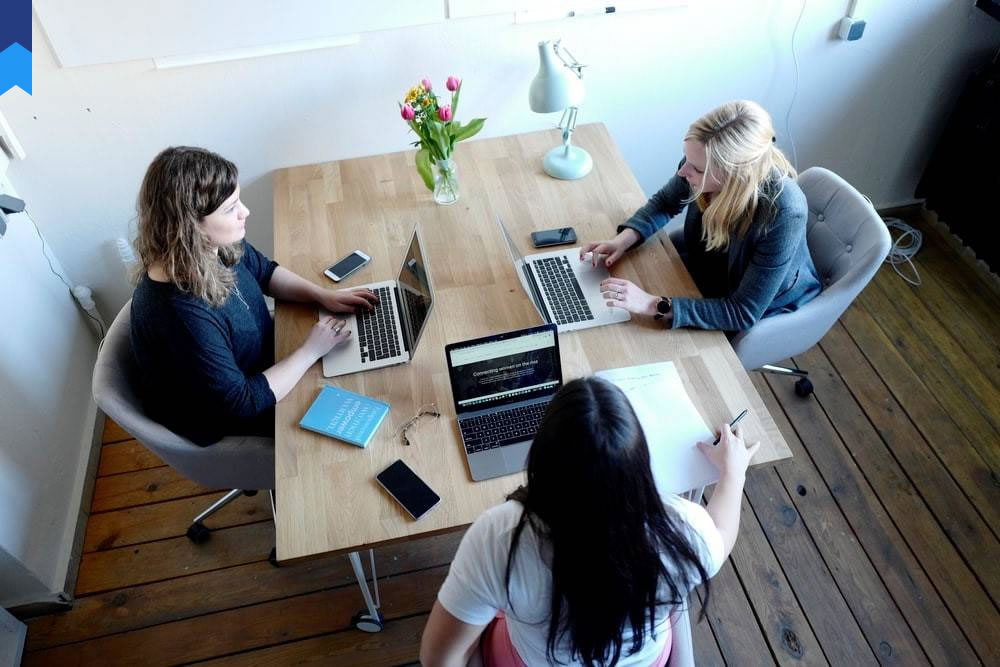
What Neuroscience Can Teach Us About Antenna Design
Antenna design, at first glance, might seem a world away from the intricacies of the human brain. However, a closer examination reveals surprising parallels between the complex signal processing in neuroscience and the challenges of optimizing antenna performance. This article explores these unexpected connections, demonstrating how principles from neuroscience can inspire innovative approaches to antenna design and overcome current limitations.
Adaptability and Dynamic Reconfiguration
The human brain is a master of adaptation. It constantly reconfigures its neural pathways in response to new information and environmental changes, a process known as neuroplasticity. This remarkable ability allows us to learn, adapt, and even recover from brain injuries. Similarly, future antennas could benefit from greater adaptability. Imagine antennas that can dynamically adjust their shape, size, and radiation patterns to optimize signal reception in ever-changing environments. This concept is already being explored through the use of metamaterials and reconfigurable antenna designs. For instance, research at MIT has yielded antennas capable of adjusting their beam direction and polarization, mimicking the brain's ability to selectively focus attention. This dynamic reconfiguration is crucial in applications such as cognitive radio, where antennas must share limited spectrum resources efficiently. A second example lies in the development of flexible, conformable antennas, which can mold themselves to different surfaces, much like the brain's ability to conform to the skull.
Consider the case of a self-driving car navigating a complex urban environment. A traditional fixed antenna might struggle with signal blockage from buildings and other vehicles. However, a neuro-inspired adaptable antenna could dynamically reconfigure its pattern to maintain a strong signal, mirroring the brain's ability to compensate for sensory input disruptions. Another application would be in the realm of satellite communication. Adaptive antennas in satellites could compensate for atmospheric disturbances and signal attenuation, ensuring stable communication links. Furthermore, the integration of artificial intelligence (AI) algorithms, inspired by neural networks, could provide real-time control and optimization of the antenna's reconfiguration strategies, adding another layer of adaptive behavior.
Neuroscience offers a valuable framework for understanding the principles of adaptive systems. By studying how the brain's neural networks adapt and learn, researchers can develop algorithms and control systems for dynamically reconfigurable antennas, potentially leading to more robust and efficient signal processing. One example of this integration is the use of spiking neural networks (SNNs) to control antenna arrays. SNNs, inspired by the brain's spiking neurons, offer the potential for energy-efficient and real-time signal processing.
The future of antenna technology lies in mimicking the brain’s extraordinary adaptability. By integrating neuro-inspired algorithms and materials, we can create antennas capable of dynamically adjusting to changing environmental conditions and optimizing signal reception in complex scenarios. This adaptability will significantly advance the capabilities of wireless communication systems in a wide range of applications.
Redundancy and Fault Tolerance
The brain’s remarkable resilience stems from its inherent redundancy. If one neural pathway is damaged, others can often compensate, ensuring the continued functioning of cognitive processes. This fault tolerance is a critical design principle that can be adopted in antenna systems to improve their reliability. Imagine a situation where an antenna array experiences a component failure. A conventional design might result in a complete system outage. However, a neuro-inspired design could incorporate redundancy, allowing the remaining elements to compensate for the failure and maintain functionality. This principle is already being applied in some antenna systems through the use of redundant components and diverse signal paths.
Consider the example of a large-scale antenna array used in radio astronomy. The failure of a single antenna element could significantly impact the overall performance. However, a system designed with redundancy would be able to maintain observational capabilities despite the failure. A similar concept applies to the communication systems of spacecraft, where redundancy is critical to ensure reliable operation during long-duration missions. Redundancy ensures continued operation even in the face of unexpected component failure.
Research into fault-tolerant systems is being actively pursued in the field of antenna design. Advanced techniques such as error-correcting codes and distributed signal processing algorithms can be incorporated to mitigate the effects of component failures. The brain also demonstrates remarkable fault tolerance through parallel processing and distributed representation of information. Mimicking these principles in antenna design could lead to systems that are less vulnerable to component failures.
By studying the brain's fault tolerance mechanisms, we can create more robust antenna systems capable of withstanding component failures and maintaining their operational capabilities. These designs will be crucial in applications where reliability is paramount, such as critical infrastructure and space communication.
Energy Efficiency and Low Power Consumption
The human brain is remarkably energy-efficient, consuming only about 20 watts of power – significantly less than many modern electronic devices. This efficiency is achieved through a combination of sophisticated signaling mechanisms and highly optimized neural network architectures. Antenna systems, especially large-scale arrays, often consume considerable power, making energy efficiency a significant design challenge. By mimicking the brain's efficient signal processing techniques, we can strive to create antennas with significantly lower power consumption.
A prime example is the development of low-power antennas for wearable devices. The need for minimal power consumption is paramount for battery-powered devices such as smartwatches and fitness trackers. Neuro-inspired designs could significantly extend the battery life of these devices by minimizing energy consumption. Similarly, low-power antennas are vital for the proliferation of Internet of Things (IoT) devices. The deployment of millions of IoT devices requires minimal energy consumption, making energy efficiency a key factor in their successful implementation.
Researchers are exploring various techniques for reducing power consumption in antenna systems. These include the use of low-power RF components, energy-efficient signal processing algorithms inspired by the brain, and novel antenna architectures that minimize energy loss. For instance, the use of highly selective antennas that only receive signals from desired sources can reduce power consumption compared to more traditional, broad-band antennas.
The brain’s energy efficiency provides a blueprint for developing next-generation antennas with significantly reduced power consumption. This efficiency will be crucial for applications requiring long operational lifetimes or operating in environments with limited power resources.
Signal Processing and Noise Reduction
The brain is constantly bombarded with sensory information, much of which is irrelevant or noisy. However, it possesses highly sophisticated mechanisms for filtering out this noise and extracting meaningful information. Antenna systems also face the challenge of dealing with noise and interference, which can degrade signal quality and limit performance. By understanding how the brain processes information in noisy environments, we can develop novel signal processing techniques for antennas. For example, the brain's ability to selectively attend to specific stimuli can inspire the design of adaptive beamforming techniques for antennas. These techniques allow antennas to focus on desired signals while suppressing interference from other sources. A notable case is the development of advanced filtering techniques for cellular base stations. These techniques, inspired by the brain’s selective attention mechanisms, can mitigate the impact of multipath interference and improve call quality.
Another example lies in the development of noise-canceling headphones. These devices use advanced signal processing algorithms to reduce unwanted background noise, mimicking the brain's ability to filter out irrelevant sensory inputs. Similar algorithms could be applied in antenna systems to improve signal-to-noise ratio (SNR) and enhance performance in noisy environments.
Neuroscience provides a valuable framework for understanding the principles of robust signal processing in the presence of noise. By studying how the brain filters out irrelevant information and extracts meaningful signals, we can develop more efficient and robust signal processing techniques for antennas, leading to better performance and improved communication quality. For instance, the use of biologically inspired algorithms such as spiking neural networks (SNNs) has shown promise in the processing of noisy signals with greater energy efficiency.
The future of antenna design will likely involve integrating advanced signal processing techniques inspired by the brain's remarkable ability to handle noise and interference. These techniques will be crucial for ensuring reliable communication in increasingly congested wireless environments.
Spatial Awareness and Beamforming
The brain possesses an exceptional ability to perceive and interpret spatial information. It uses this ability to navigate environments, interact with objects, and understand the world around us. This spatial awareness is crucial for creating antenna systems that can effectively transmit and receive signals in complex environments. By understanding how the brain processes spatial information, we can design more effective beamforming techniques for antenna arrays. Beamforming is a technique that allows multiple antennas to focus their signals in a specific direction, enhancing signal strength and reducing interference. Neuro-inspired beamforming algorithms could adapt to the environment dynamically, optimizing signal transmission and reception in real-time, mirroring the brain's dynamic spatial awareness.
A clear example of this is in the use of antenna arrays in 5G cellular networks. These arrays use beamforming techniques to direct signals toward specific mobile devices, improving signal quality and data throughput. Neuro-inspired beamforming algorithms could further enhance the performance of these systems by adapting to changing environments and optimizing signal transmission in real-time.
Another application lies in the development of high-resolution radar systems. By using multiple antennas and advanced beamforming techniques, these systems can create detailed images of their surroundings. Neuro-inspired algorithms could improve the resolution and accuracy of these systems by optimizing the signal processing and beamforming strategies.
The brain’s spatial awareness is a powerful source of inspiration for future antenna systems. By integrating neuro-inspired algorithms and techniques, we can develop antenna arrays capable of dynamically adapting to changing environments and optimizing signal transmission and reception, leading to significant improvements in wireless communication systems.
Conclusion
The seemingly disparate fields of neuroscience and antenna design share a surprising number of common challenges and opportunities. By drawing inspiration from the brain's remarkable abilities in adaptation, redundancy, energy efficiency, signal processing, and spatial awareness, we can create new generations of antennas that are more efficient, robust, and adaptable than ever before. This interdisciplinary approach offers a powerful pathway to overcome current limitations in antenna technology and unlock significant advances in wireless communication systems. The future of antenna design is likely to be shaped by this powerful combination of biological inspiration and technological innovation. Further research into these parallels holds immense potential for revolutionizing wireless communication.